6.6 Classification of Mass Wasting
Steven Earle and Laura J. Brown
It’s important to classify slope failures to understand what causes them and learn how to mitigate their effects. The three criteria used to describe slope failures are:
- The type of material that failed (typically either bedrock or unconsolidated sediment)
- The mechanism of the failure (how the material moved)
- The rate at which it moved
The type of motion is the most important characteristic of a slope failure, and there are three different types of motion:
- If the material drops through the air, vertically or nearly vertically, it’s known as a fall.
- If the material moves as a consolidated mass along a sloping surface, it’s a slide.
- If the material is unconsolidated, it moves like a fluid; it’s a flow.
Unfortunately, it’s not normally that simple. Many slope failures involve two of these types of motion, some involve all three, and in many cases, it’s not easy to tell how the material moved. The types of slope failure that we’ll cover here are summarized in Table 6.6.1.
[Skip Table] | |||
Failure Type | Type of Material | Type of Motion | Rate of Motion |
---|---|---|---|
Rockfall | Rock fragments | Vertical or near-vertical fall (plus bouncing in many cases) | Very fast (Greater than 10s of metres per second) |
Rockslide | A large rock body | Motion as a unit along a planar surface (translational sliding) | Typically very slow (millimetres per year to centimetres per year), but some can be faster |
Rock avalanche | A large rock body that slides and then breaks into small fragments | Flow (at high speeds, the mass of rock fragments is suspended on a cushion of air) | Very fast (Greater than tens of metres per second) |
Creep or solifluction | Soil or other overburden; in some cases, mixed with ice | Flow (although sliding motion may also occur) | Very slow (millimetres per year to centimetres per year) |
Slump | Thick deposits (a metre to 10s of metres) of unconsolidated sediment | Motion as a unit along a curved surface (rotational sliding) | Slow (centimetres per year to metres per year) |
Mudflow | Loose sediment with a significant component of silt and clay | Flow (a mixture of sediment and water moves down a channel) | Moderate to fast (centimetres per second to metres per second) |
Debris flow | Sand, gravel, and larger fragments | Flow (similar to a mudflow, but typically faster) | Fast (metres per second) |
Rock Fall
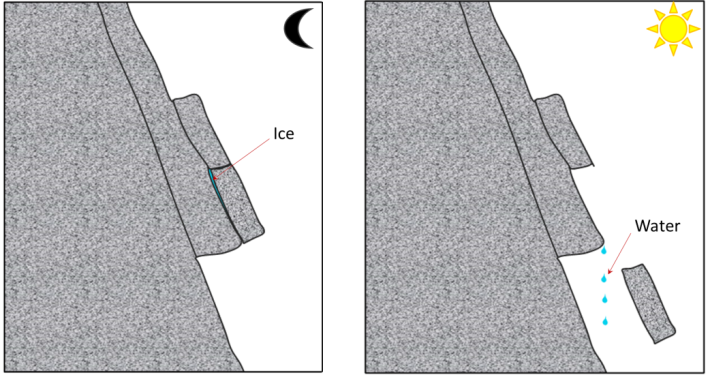
Rock fragments can break off relatively easily from steep bedrock slopes, most commonly due to frost-wedging in areas where there are many freeze-thaw cycles per year. If you’ve ever hiked along a steep mountain trail on a cool morning, you might have heard the occasional fall of rock fragments onto a talus slope. This happens because the water between cracks freezes and expands overnight, and then when that same water thaws in the morning sun, the fragments that had been pushed beyond their limit by the ice, fall to the slope below (Figure 5.5.1).
A typical talus slope near Keremeos in southern B.C. is shown in Figure 5.5.2. In December 2014, a large block of rock split away from a cliff in this same area. It broke into smaller pieces that tumbled down the slope and crashed into the road, smashing the concrete barriers and gouging out large parts of the pavement. Luckily no one was hurt.
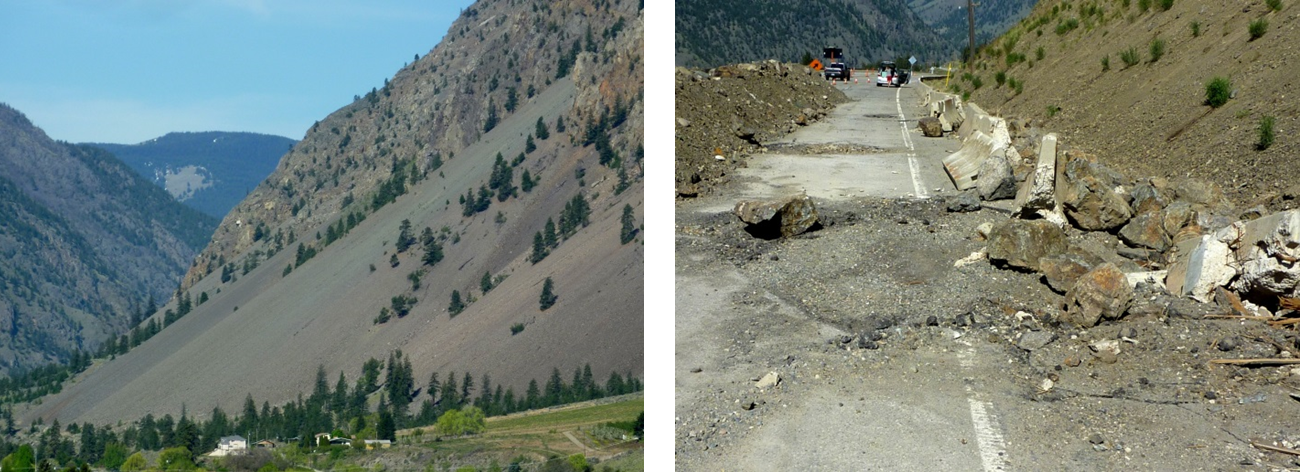
Rock Slide
A rock slide is the sliding motion of rock along a sloping surface. In most cases, the movement is parallel to a fracture, bedding, or metamorphic foliation plane, and it can range from very slow to moderately fast. The word sackung describes the very slow motion of a block of rock (millimetres per year to centimetres per year) on a slope. A good example is the Downie Slide north of Revelstoke, B.C., which is shown in Figure 5.5.3. In this case, a massive body of rock is very slowly sliding down a steep slope along a plane of weakness that is approximately parallel to the slope. The Downie Slide, which was first recognized in the 1950s, before the construction of the Revelstoke Dam in the late 1970s, was moving very slowly at the time (a few centimetres per year). Geological engineers were concerned that the presence of water in the reservoir (visible in Figure 5.5.3) could further weaken the plane of failure, leading to an acceleration of the motion. The result would have been a catastrophic failure into the reservoir that would have sent a wall of water over the dam and into the community of Revelstoke. During the dam’s construction, they tunnelled into the rock at the base of the slide and drilled hundreds of drainage holes upward into the plane of failure. This allowed water to drain out so that the pressure was reduced, which reduced the rate of movement of the sliding block. BC Hydro monitors this site continuously; the slide block is currently moving more slowly than it was prior to the construction of the dam.
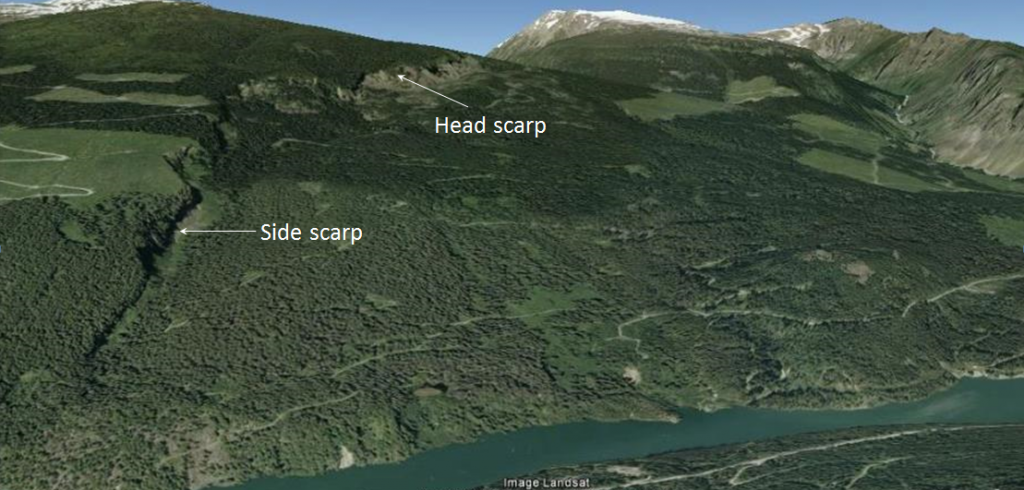
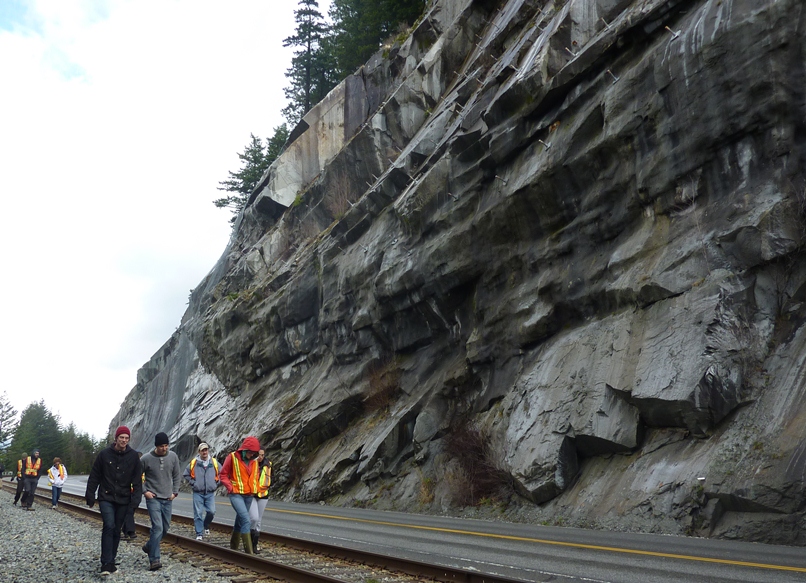
In the summer of 2008, a large block of rock slid rapidly from a steep slope above Highway 99 near Porteau Cove (between Horseshoe Bay and Squamish). The block slammed into the highway and adjacent railway and broke into many pieces. The highway was closed for several days, and the slope was subsequently stabilized with rock bolts and drainage holes. As shown in Figure 5.5.4, the rock is fractured parallel to the slope, and this almost certainly contributed to the failure. However, it is not actually known what triggered this event as the weather was dry and warm during the preceding weeks, and there was no significant earthquake in the region.
Rock Avalanche
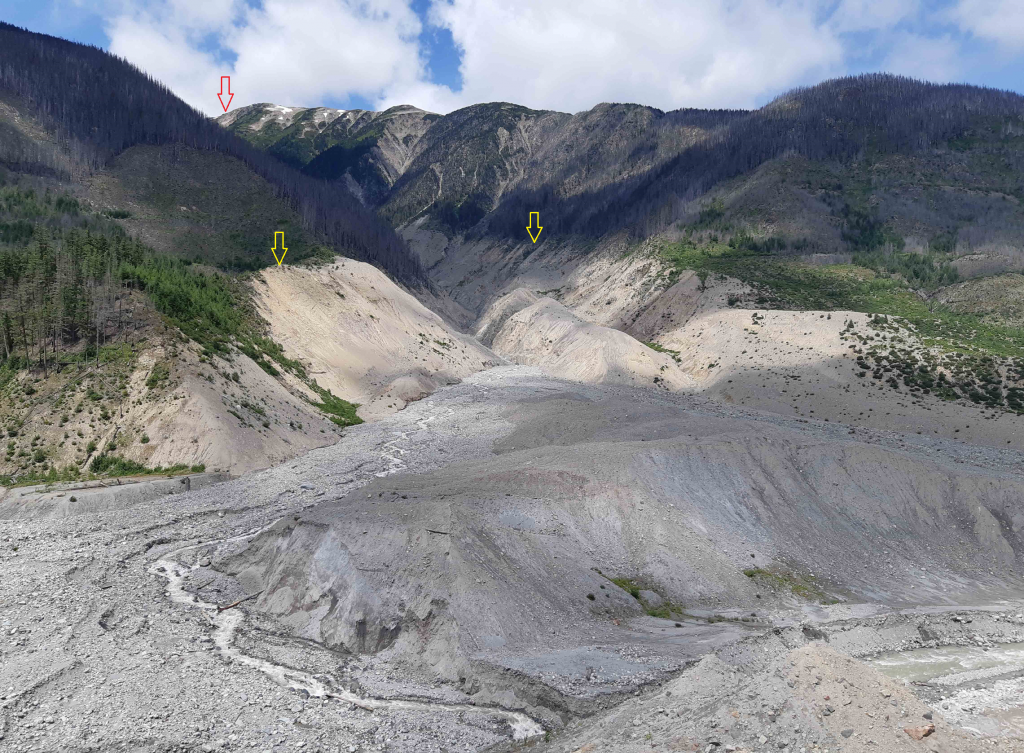
If a rock slides and then starts moving quickly (metres per second), the rock is likely to break into many small pieces. At that point, it turns into a rock avalanche, in which the large and small fragments of rock move in a fluid manner supported by a cushion of air within and beneath the moving mass. The 1965 Hope Slide (Figure 5.2) was a rock avalanche, as was the famous 1903 Frank Slide in southwestern Alberta.
Creep or Solifluction
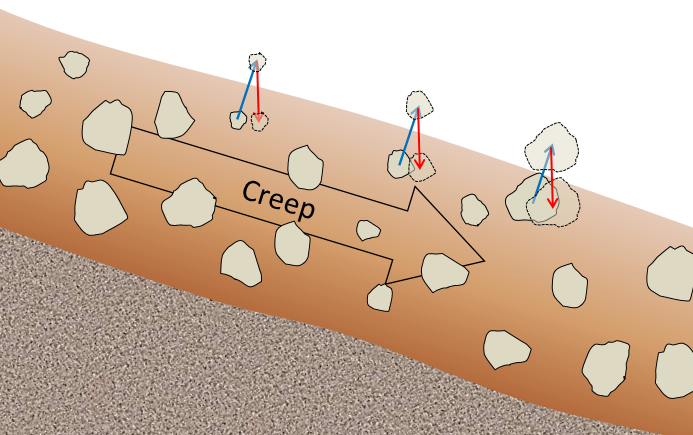
The very slow—millimetres per year to centimetres per year—movement of soil or other unconsolidated material on a slope is known as creep. Creep, which normally only affects the upper several centimetres of loose material, is typically a type of very slow flow, but in some cases, sliding may take place. Creep can be facilitated by freezing and thawing because, as shown in Figure 5.5.6, particles are lifted perpendicular to the surface by the growth of ice crystals within the soil and then let down vertically by gravity when the ice melts. The same effect can be produced by frequent wetting and drying of the soil. In cold environments, solifluction is a more intense form of freeze-thaw-triggered creep.
Creep is most noticeable on moderate-to-steep slopes where trees, fence posts, or grave markers are consistently leaning in a downhill direction. In the case of trees, they try to correct their lean by growing upright, leading to a curved lower trunk known as a “pistol butt.” An example is shown in Figure 5.5.7.
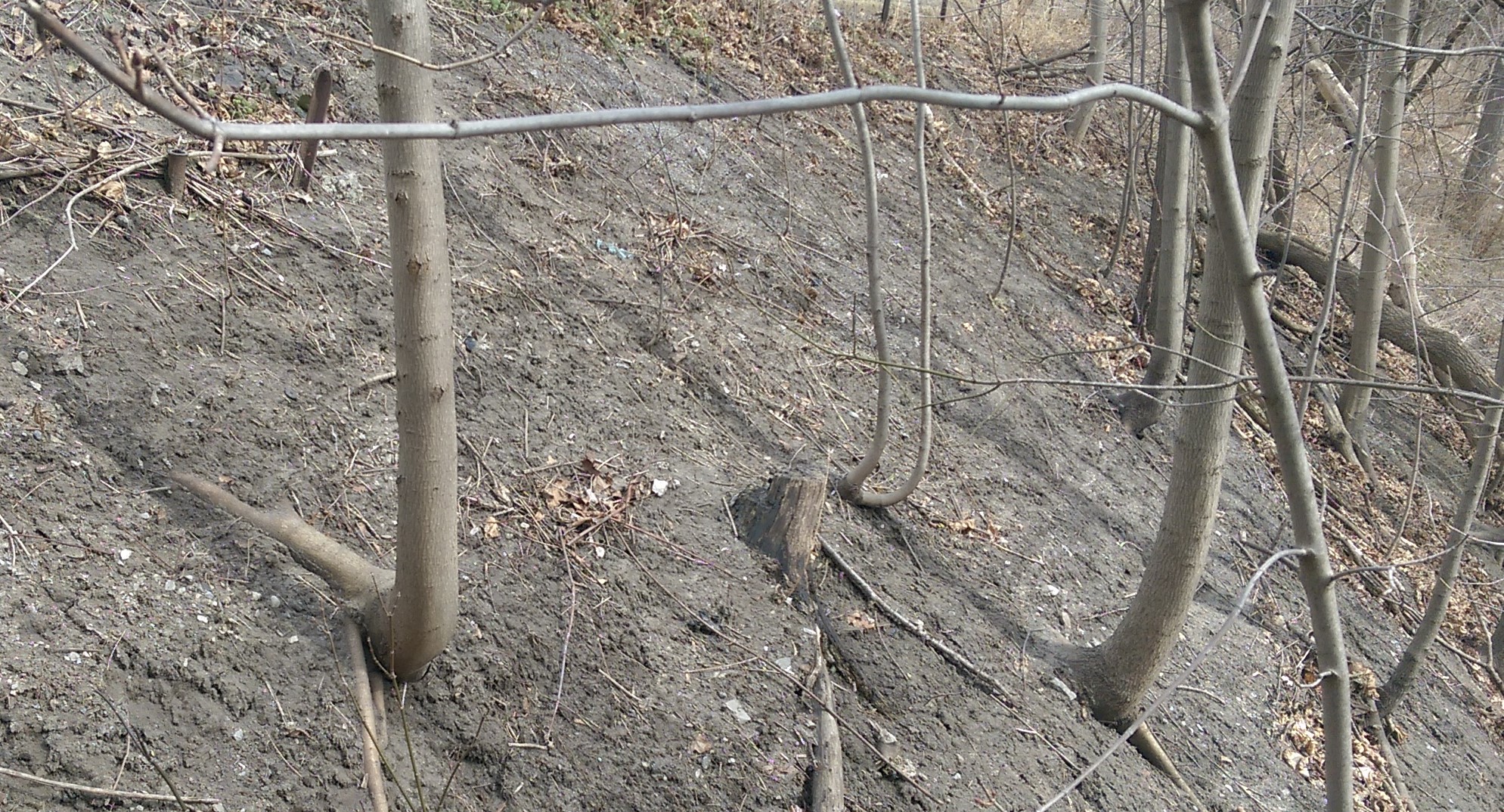
Slump
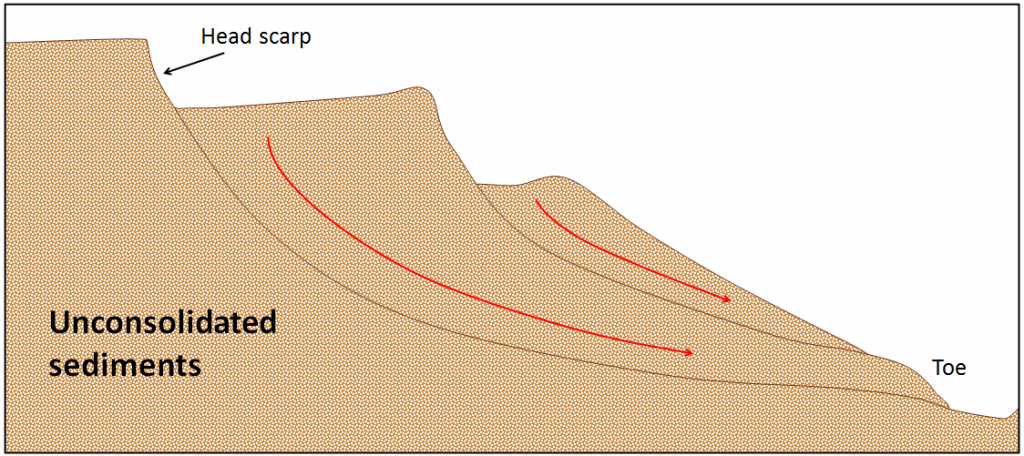
A slump is a type of slide (movement as a consolidated mass) that takes place within thick unconsolidated deposits (typically thicker than 10 metres). Slumps involve movement along one or more curved failure surfaces, with downward motion near the top and outward motion toward the bottom (Figure 5.5.8). They are typically caused by an excess of water within these materials on a steep slope.
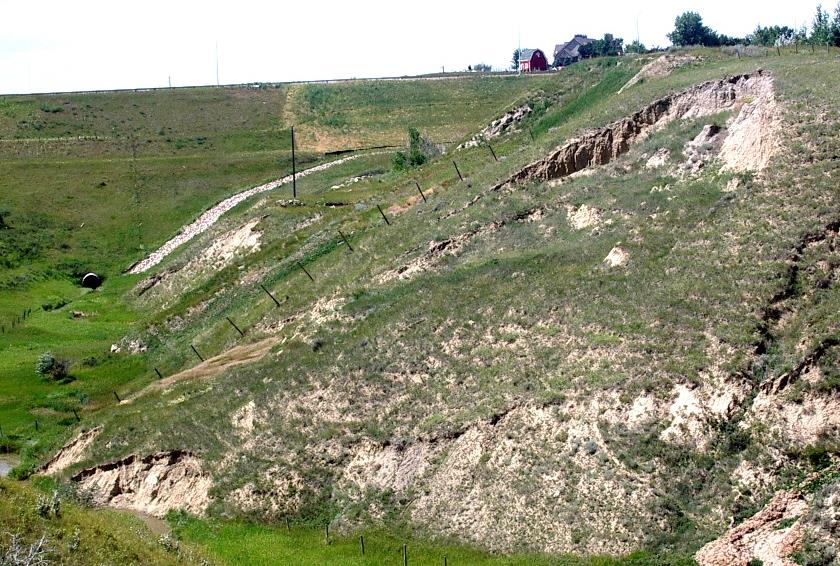
An example of a slump in the Lethbridge area of Alberta is shown in Figure 5.5.9. This feature has likely been active for many decades and moves a little more whenever there are heavy spring rains and significant snowmelt runoff. The toe of the slump is failing because it has been eroded by the small stream at the bottom.
Mudflows and Debris Flows
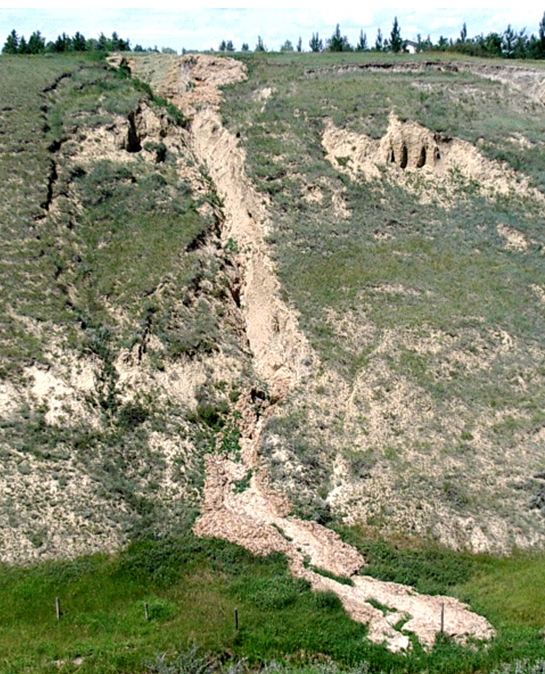
As you saw in Exercise 5.4.1, when a mass of sediment becomes completely saturated with water, the mass loses strength, to the extent that the grains are pushed apart, and it will flow, even on a gentle slope. This can happen during rapid spring snowmelt or heavy rains and is also relatively common during volcanic eruptions because of the rapid melting of snow and ice. (A mudflow or debris flow on a volcano or during a volcanic eruption is a lahar.) If the material involved is primarily sand-sized or smaller, it is known as a mudflow, such as the one shown in Figure 5.5.10.
If the material involved is gravel-sized or larger (boulders, trees, parts of buildings, anything in its path that is picked up and carried downslope), it is known as a debris flow. Because it takes more gravitational energy to move larger particles, a debris flow typically forms in an area with steeper slopes and more water than does a mud flow. In many cases, a debris flow occurs within a steep stream channel, and is triggered by the collapse of bank material into the stream. This creates a temporary dam and then a major flow of water and debris when the dam breaks. This situation led to the fatal debris flow at Johnsons Landing, B.C., in 2012. A typical west-coast debris flow is shown in Figure 5.5.11. This event took place in November 2006 in response to very heavy rainfall. There was enough energy to move large boulders and to knock over large trees.
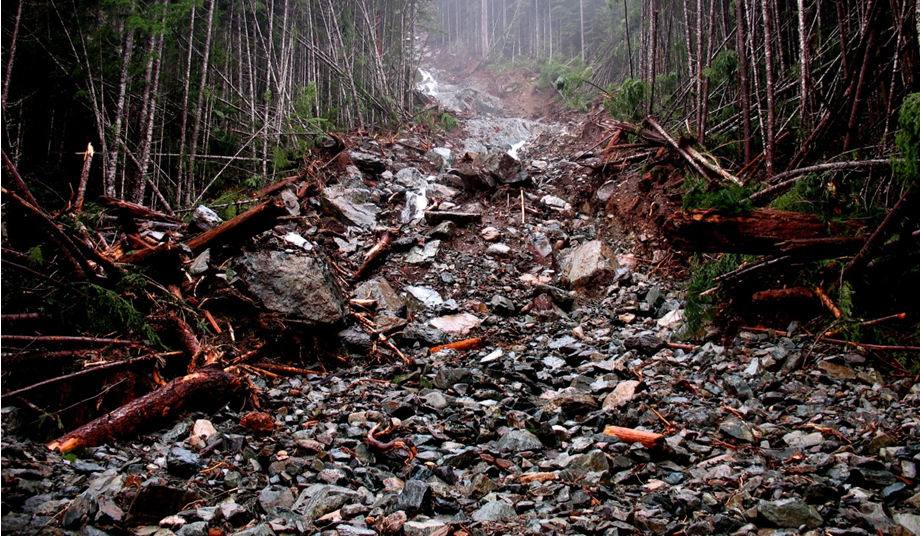
Exercise 5.4.2 Classifying slope failures
Media Attributions
- Figure 5.5.1, 5.5.2: © Steven Earle. CC BY.
- Figure 5.5.3: © Google Earth. Used with permission for non-commercial purposes.
- Figure 5.5.4: © Steven Earle. CC BY.
- Figure 5.5.5: “2010 Mt. Meager rock avalanche” © Isaac Earle. CC BY.
- Figure 5.5.6, 5.5.7, 5.5.8, 5.5.9, 5.5.10, 5.5.11, 5.5.12: © Steven Earle. CC BY.