Unit 2c. Physical Properties of Milk
In this chapter we will cover the following physical properties. More information can be found in Walstra‘s text (avalilable in ARES). Another easy-to-read and useful material to access is the Tetrapack’s Dairy Processing Handbook.
Main Physical properties of milk:
- Density
- Viscosity
- Freezing Point
- Optical Properties
- Acid-base Equilibria
The density of milk and milk products is used for the following;
- to convert volume into mass and vice versa
- to estimate the solids content
- to calculate other physical properties (e.g. kinematic viscosity)
Density, the mass of a certain quantity of material divided by its volume, is dependent on the following:
- temperature at the time of measurement
- temperature history of the material
- composition of the material (especially the fat content)
- inclusion of air (a complication with more viscous products)
With all of this in mind, the density of milk varies within the range of 1027 to 1033 kg /m3 at 20° C.
The following table gives the density of various fluid dairy products as a function of fat and solids-not-fat (SNF) composition:
Product Composition | Density (kg/L) at: | |||||
---|---|---|---|---|---|---|
Product | Fat (%) | SNF (%) | 4.4°C | 10°C | 20°C | 38.9°C |
Producer milk | 4 | 8.95 | 1.035 | 1.033 | 1.03 | 1.023 |
Homogenized milk | 3.6 | 8.6 | 1.033 | 1.032 | 1.029 | 1.022 |
Skim milk, pkg | 0.02 | 8.9 | 1.036 | 1.035 | 1.033 | 1.026 |
Fortified skim | 0.02 | 10.15 | 1.041 | 1.04 | 1.038 | 1.031 |
Half and half | 12.25 | 7.75 | 1.027 | 1.025 | 1.02 | 1.01 |
Half and half, fort. | 11.3 | 8.9 | 1.031 | 1.03 | 1.024 | 1.014 |
Light cream | 20 | 7.2 | 1.021 | 1.018 | 1.012 | 1 |
Heavy cream | 36.6 | 5.55 | 1.008 | 1.005 | 0.994 | 0.978 |
Viscosity
Viscosity of milk and milk products is important in determining the following:
- the rate of creaming
- rates of mass and heat transfer
- the flow conditions in dairy processes
Milk and skim milk, excepting cooled raw milk, exhibit Newtonian behaviour, in which the viscosity is independent of the rate of shear. The viscosity of these products depends on the following:
- Temperature:
- cooler temperatures increase viscosity due to the increased voluminosity of casein micelles
- temperatures above 65° C increase viscosity due to the denaturation of whey proteins
- pH: an increase or decrease in pH of milk also causes an increase in casein micelle voluminosity
Cooled raw milk and cream exhibit non-Newtonian behavior in which the viscosity is dependent on the shear rate. Agitation may cause partial coalescence of the fat globules (partial churning) which increases viscosity. Fat globules that have under gone cold agglutination, may be dispersed due to agitation, causing a decrease in viscosity. Viscosity is a very important physical properties in processing steps that involve mass and heat transfer light pumping, or pasteurizing.
Freezing Point
Freezing point depression is a colligative property which is determined by the molarity of solutes rather than by the percentage by weight or volume. In the dairy industry, freezing point of milk is mainly used to determine added water but it can also been used to determine lactose content in milk, estimate whey powder contents in skim milk powder, and to determine water activity of cheese. The freezing point of milk is usually in the range of -0.512 to -0.550° C with an average of about -0.522° C.
Correct interpretation of freezing point data with respect to added water depends on a good understanding of the factors affecting freezing point depression. With respect to interpretation of freezing points for added water determination, the most significant variables are the nutritional status of the herd and the access to water. Under feeding causes increased freezing points. Large temporary increases in freezing point occur after consumption of large amounts of water because milk is iso-osmotic with blood. The primary sources of non-intentional added water in milk are residual rinse water and condensation in the milking system.
In the following video you can watch how the freezing point is measured through Cryoscopy at the University of Guelph, AgriFood Lab.
Optical properties
Optical properties provide the basis for many rapid, indirect methods of analysis such as proximate analysis by infrared absorbency or light scattering. Optical properties also determine the appearance of milk and milk products. Light scattering by fat globules and casein micelles causes milk to appear turbid and opaque. Light scattering occurs when the wave length of light is near the same magnitude as the particle. Thus, smaller particles scatter light of shorter wavelengths. Skim milk appears slightly blue because casein micelles scatter the shorter wavelengths of visible light (blue) more than the red. The carotenoid precursor of vitamin A, ß -carotene, contained in milk fat, is responsible for the ‘creamy’ colour of milk. Riboflavin imparts a greenish colour to whey.
Refractive index (RI) is defined as the ratio of the speed of light in a vacuum to its speed in that substance. This change is related to the bending of the direction of the light once it enters the substance, and the refractive index is a measurement of this bending. Since each component of milk contributes to its refractive index, refractive index is different in milks with different origin (breed or specie) or processing. RI is normally determined at 20° C with the D line of the sodium spectrum. The refractive index of milk is 1.3440 to 1.3485 and can be used to estimate total solids.
Acid-base equilibria
Both titratable acidity and pH are used to measure milk acidity. The pH of milk at 25° C normally varies within a relatively narrow range of 6.5 to 6.7. The normal range for titratable acidity of herd milks is 13 to 20 mmol/L. Because of the large inherent variation, the measure of titratable acidity in milk per se has little practical value except to measure changes in acidity (eg., during lactic fermentation) . So we measure TA when ripenning milk for cheesemaking, since the change in pH during this time is very small but the changes in lactic acid can be measure through TA.
Concepts of Acidity and pH
All aqueous systems (including the water in you and in cheese) obey the following relationship between the concentration of hydrogen ions (H+) and hydroxyl ions (OH-). Note: the square brackets indicate concentration in moles per litre. A mole is 6 x 1023 molecules, that is, the numeral six with 23 zeros after it.
Because the actual concentrations in moles per litre are small, it is customary to express the values as exponents. For example, if we know that the concentration of hydrogen ions [H+] in a sample of milk is 0.000001 moles/L which is equivalent to 10-6 moles/L, we can calculate the concentration of hydroxyl ions as 10-14/10-6 = 10-8 moles/L which is the same as 0.00000001 moles/L. Similarly, if [H+] is 10-7, we can calculate that [OH-] is also 10-7.
It follows then that:
Key Takeaways
- Chemicals which contribute H+ or absorb OH- are acids
- Bases contribute OH- or absorb H+
The concept of pH evolved as a short hand method to express acidity. We have already seen that a hydrogen ion concentration of 0.000001 moles/L can be expressed as [1 x10-6], an expression which defines both the unit of measurement (the square bracket means concentration in moles/L) and the numerical value. The concept of pH is a further abbreviation which expresses the concentration of hydrogen ions as the negative log of the hydrogen ion concentration in units of moles/L, which is expressed by the following equation:
This sounds complex but is quite easy to apply. For example, the log10 of hydrogen ion concentration of [10-6] is equal to -6. The final step is to take the negative of the log (-1 x -6 = 6). So, 0.0000001 moles/L = [10-6] moles/L = pH 6. Using equation 1 above, if the concentration of either OH- or H+ is known, it is always possible to calculate the concentration of the other. So, if the pH of a solution is 6, the pOH is 14 – 6 = 8. Because this relationship is understood (well, OK, at least understood by the chemists!), the convention is to only report pH.
Note: that because the negative sign was dropped for convenience, decreasing pH values mean increasing acidity, or increasing concentration of H+ ions. So, although TA and pH are both measures of acidity, pH decreases with increasing acidity.
All of this can be summarized with a description of the pH scale. The pH scale for most practical purposes is from 1 to 14, although pH less than one is theoretically and practically possible
For simple acids dissolved in water as the concentration increase the acidity increases, which means the pH decreases proportionally. But because pH is a relation between H+ and OH-, and many substances are able to buffer (interact with) these H+ or OH+, so that the the addition of these acids or bases to that product won’t create linear proportional increases or decreases in the pH. That is what we call Buffer Capacity of a substance, in our case Milk. The bigger the buffer capacity, the more lactic acid is required to decrease the pH to a target level (e.g from 6.7 to 5.2).
Buffer Capacity
Buffer capacity is the ability of an aqueous system, such as milk, to resist changes in pH with addition of acids (added H+) or bases (added OH-). Specifically, buffer capacity is the amount of acid or base required to induce a unit change in pH. For example, a small addition of acid in distilled water will cause a large reduction in pH. The same amount of acid would have a small effect on the pH of milk, because milk proteins and salts neutralize the acidity. The two most important buffer components of milk are caseins ([pb glossary id=”1092″]buffer maximum[/pb glossary] near pH 4.6 – 5.0 and calcium phosphates with a large buffer maximum in milk near pH 5.0. The overlapping buffer maxima of CaP and casein in near pH 5.0 is extremely important to cheese manufacture because the optimal minimum pH for most cheese is in the range of 5.0 – 5.3. That is the desired minimum pH during the first few days after manufacture is 5.0 – 5.3 for most firm to hard varieties. As the pH of cheese is reduced towards pH 5.0 by lactic acid fermentation, the buffer capacity is increasing (i.e., each incremental decrease in pH requires more lactic acid). The effect is to give the cheese maker considerable room for variation in the rate and amount of acid production. Without milk’s built-in buffers, it would be difficult to produce cheese in the optimum pH range.
To make it easier to understand the buffering capacity of milk, we have plotted an acid titration (with HCL) of cows milk from 6.5 (around natural pH in milk) to pH 2. The units on the y axis are known as buffer capacity. But for the purpose of this unit we will think of it as the amount of acid required to decrease the pH. The green shape represents the amount of acid required to bring the pH from 6.5 to 6.1 (0.4 units of pH). This is the around the same decrease of milk pH that we require to be able to drain the whey when making cheddar. Now lets look at the second shape (light blue). That represents the amount of acid required to bring the pH from 5.8 to 5.4 (0.4 units). On the example of cheddar 5.4 would be around the time we are able to mill the curd. Note that the amount of acid required is around double the amount.
Why is this?
Because the buffer capacity of milk peaks around pH 5. This is due mainly due to the solubilization of calcium phosphate from casein which buffer those extra H+ from the acid. There are other peaks in the curve which we we don’t need to discuss now.
The next graph shows the same data but comparing cows (in red), goats (green) and sheep milk (blue). This sheep milk has approximately two times the protein that the cow’s milk and a higher casein/whey protein ratio. Because of the extra caseins in sheep milk, there is an extra amount of acid needed to produce the same decrease in pH. If we were comparing cows milk with 2 times ultrafiltered milk (concentrated) we would see similar data.
Note: this is an aside to the chemists in the crowd who could correctly argue that CaP has three buffer maxima at pH 2.1, 7.2, and 12.5. That’s true in water. In milk only the buffer maximum at pH 7.2 is of interest., and, importantly, because CaP is complexed with citrates and caseins in the casein micelle, the pH 7.2 maximum is reduced to the region of 5.2.
Titratable Acidity, pH and cheesemaking
Tritratable acidity (TA) and pH are both measures of acidity but, for most purposes, pH is a better process control tool because the pH probe measures only those H+ that are free in solution and not associated with salts or proteins. This is important because it is the free H+ ions that directly affect quality parameters such as sour taste and protein functionality. The pH also directly affects microbial growth and survival, so pH, rather than TA is the best indicator of the preservation and safety effects of acidity. pH is the most important factor available to the cheese maker to control spoilage and pathogenic organisms. Accordingly, the pH history of the milk and cheese or whey is important troubleshooting information. Cheese moisture, mineral content, texture, and flavour development are all influenced directly by pH.
In contrast, TA measures not only the free hydrogen ions, but also hydrogen ions associated with organic acids and proteins. This means, for example, that TA of fresh good quality milk varies from about 0.12 to about 0.25% lactic acid equivalents depending mainly on the protein content in the milk. However, the pH of fresh good quality milk will always be in the range of 6.6 to 6.8, which is the same pH as the blood of all mammals. Similar differences occur during cheese manufacture; the pH gives a true indication of acid development during the entire process, so the optimum pH at each step is independent of other variables. However, the optimum TA at each step in cheese making will vary with initial milk composition, milk heat treatments, and the procedure used to standardize milk composition.
An illustration of the difference between TA and pH is the effect of cutting. Up to the time of cutting, TA of the milk increases with the development of acidity by the culture. After cutting, the TA of the whey is much lower. This does not mean that acid development stopped, but that titratable H+ ions associated with the milk proteins are not present in the whey. Again, this is about buffer capacity. The effect of protein removal on the TA of whey is related to the ability of protein and milk salts to “buffer” the milk against changes in pH. That same buffering property is the reason it helps to take acidic medication, like aspirin, with milk.
Note: that there is practically no lactic acid in fresh milk, but it is customary to report TA in terms of % lactic acid because the difference in TA during cheese making is entirely due to production of lactic acid. That is, the original TA in milk is not because of lactic acid, but the increase of TA during, for example, ripening of milk is a result of lactic acid production.
Application Notes for cheese
As described in section 2.4.1, both titratable acidity (TA) and pH are measures of acidity. For most process control purposes, pH is a more useful measurement. Some cheese makers, however, still use TA to monitor initial acid development (that is, to check for culture activity) during the first hour after adding the culture. For this purpose, TA is a more reliable indicator because relative to pH measurement, it is more sensitive to small changes in milk acidity.
In modern dairy fermentations, TA is also being used along with pH to better determine the amount and type of cultures to be used for high protein substrates. For example, fermentation of UF milk retentates.
pH Measurement
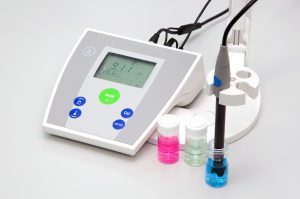
If you want to know how to measure pH and TA, please check the three videos posted on unit 2d on courselink.
Notes for dairy:
The pH of cheese milk, whey, and soft cheese can be measured directly. Firm and hard cheese must be fragmented before analysis.
Always measure cheese pH in duplicate and use care in handling the electrode.
Place the fragmented cheese in a 30 ml vial or a small beaker and gently push the electrode into the cheese – too much haste is likely to break the electrode on the bottom of the beaker. To ensure good contact, press the cheese around the electrode with your fingers. There is no need to rinse the electrode between cheese samples. However, if the electrode is stored in buffer it should be rinsed with water before measuring cheese pH.
Always store the electrode in pH 4 buffer or in a special potassium chloride solution designed for that purpose. Do not rub the electrode. The electrode should be washed with detergent and rinsed with acetone occasionally to remove fat and protein deposits.
Since it is difficult to measure cheese directly with a protected electrode, an alternative to packing fragmented cheese around an electrode is to stir fragmented cheese in some distilled water and measure the pH of the mixture.
When using TA to monitor initial culture activity, note that:
- You are looking for a measurable increase in TA to confirm that the culture is active.
- Different people will interpret the coloured endpoint differently, so it is important that the same person takes both the initial and final TA measurements.
- The principal divisions on most acidimeters are units of 0.1% lactic acid with subdivisions corresponding to 0.01% lactic acid. It is possible to interpolate between the subdivisions to obtain readings to the third decimal place. In practice, for most analysts, the sensitivity is about 0.005% lactic acid. That means it is possible to reliably measure a change in TA of 0.005% lactic acid. So, if the TA increase is greater than 0.005%, you can conclude that the culture is active. In most cases, TA increases in the range of 0.005% to 0.010% are obtained after 30-60 minutes of ripening (that is, 30-60 minutes after adding the lactic cultures).