7a: Energy in Organics
Written by Jenna West
Introduction
Energy flows in organic agriculture incorporate many of the topics discussed in previous sections through interwoven cycles (Figure 1). Photosynthesis is the most obvious of these cycles, as plants take solar energy and use it to increase their biomass, which humans then harvest and eat as food. Other energy, like fuel and human labour, are direct and indirect energy inputs that facilitate the interception and usage of solar energy by crop plants. This chapter examines how photosynthesis occurs from the scale of a leaf to the crop level. Factors that affect photosynthetic productivity and how solar energy usage could be improved in organic agriculture will also be explored. We will then look at direct and indirect human and fossil fuel energy inputs of organic agriculture. The great debate on energy efficiency in organic and conventional agriculture will then be introduced and summarized from current literature on the topic. Developments in energy efficiency will be used to project how shifts in energy inputs and how we think about energy use efficiency could create positive change in the sustainability of the agri-food system.
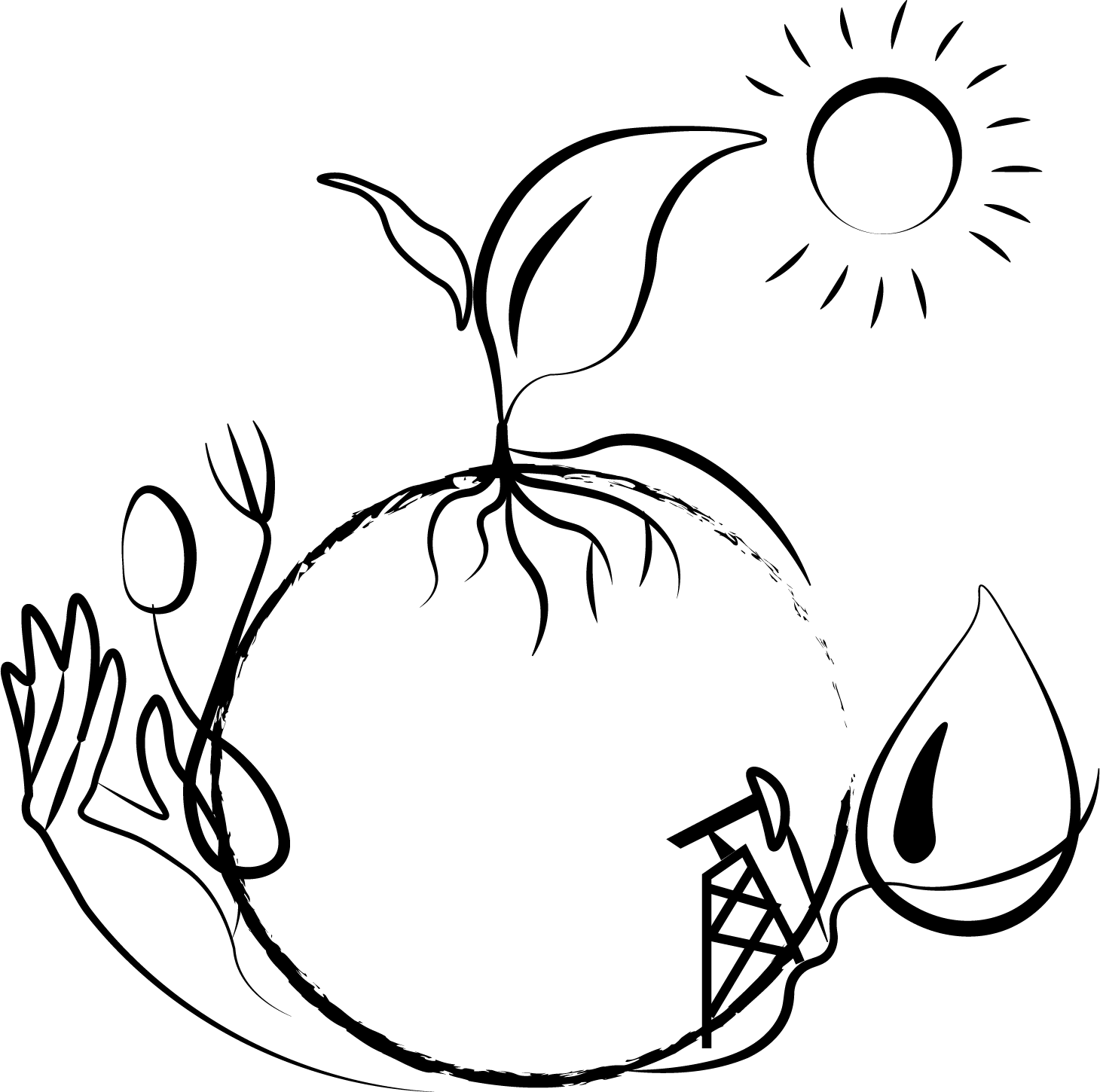
Solar Energy
Photosynthesis of a Leaf
At the leaf level, the pigment chlorophyll, located in the chloroplasts of the leaf, absorbs the sun’s light energy. Chlorophyll absorbs light in the 400-700nm range. This light in the blue and red regions of the light spectrum, as shown in Figure 2, is used for photosynthesis, and is thus called PAR (photosynthetically active radiation).
Improving Solar Energy Usage
Maximizing the use of solar energy in organic agriculture is a strategy to increase the efficiency of the system. Breeding crops that produce full canopies early in the season and stay green as late into the season as possible ensures the maximum amount of solar energy is intercepted and utilized by the crop. Extending the growing season using adapted cultivars, greenhouses, hoop houses and row covers are other ways to make use of solar energy early and late in the growing season, especially in mild climates near the Great Lakes and in the coastal regions of Canada.
Besides manipulating the length of the growing season, harvesting solar energy for power is another method to increase usage. Agrivoltaics is the term for using land for agriculture and photovoltaics simultaneously (Pascaris et al. 2021). This can be done by interspersing solar panels with crops, or in livestock grazing fields (Pascaris et al. 2021). The largest barrier found through research regarding the increase of agrivoltaics is acceptance by farmers (Pascaris et al. 2020). Creating a flexible system for different farm types and scales with proper compensation and ensuring installing solar panels is a wise long-term decision for agricultural systems where good strategies increase acceptance by farmers (Pascaris et al. 2020). A recent study on lambs foraging in systems with and without photovoltaic panels found systems with panels had a 9-33% reduction in forage biomass production due to shade and heavy traffic by lambs in shaded areas (Andrew et al. 2021). Despite this, the quality of forage was improved due to a variety of reasons, and as such, lamb production was similar across the systems (Andrew et al. 2021). Additionally, as shade provision becomes increasingly important in animal welfare policies, panels take on the dual purpose of providing shade to decrease livestock heat stress, while also creating power from solar energy.
Fossil Fuel and Human Energy
Energy Inputs in Organics
The largest source of energy in organic farming is solar; however, since this energy source is considered free and limitless, it is rarely considered when studying the energy efficiency of organic agricultural systems. Human labour is also key in organic agriculture; however, it is difficult to measure compared to other energy inputs and so is left out of many analyses (Shepherd et al. 2003). It is worth noting that the mechanization of agriculture has led to a decrease in the amount of human labour required to grow many crops (Pimentel 2009). For example, corn produced by humans and draft power requires 1,200 hours of labour per hectare produced, while modern technology has reduced this to 11 hours/ha (Pimentel 2009). Compared to conventional agriculture, certain organic agriculture can have increased manual labour requirements, especially in horticultural systems.
The rest of the energy inputs in organic agriculture are sorted into direct and indirect categories as seen in Table 1 below. Fuel and electricity are direct inputs. Fuel for tractors decreases in organic agriculture due to the absence of pesticide applications but can increase due to increased mechanical weeding, especially if flame weeders are used (Shepherd et al. 2003). Additionally, compost is heavier and less nutrient dense than synthetic nitrogen fertilizer, so additional fuel for tractors is required to spread compost even though it reduces indirect energy inputs (Shepherd et al. 2003). Indirect energy uses consist of the manufacturing and maintenance of equipment and the transport of compost. The energy input required for manufacturing and transporting pesticides and synthetic nitrogen fertilizer is not included in organic agriculture and is the most significant difference in energy usage between conventional and organic agriculture (Shepherd et al. 2003).
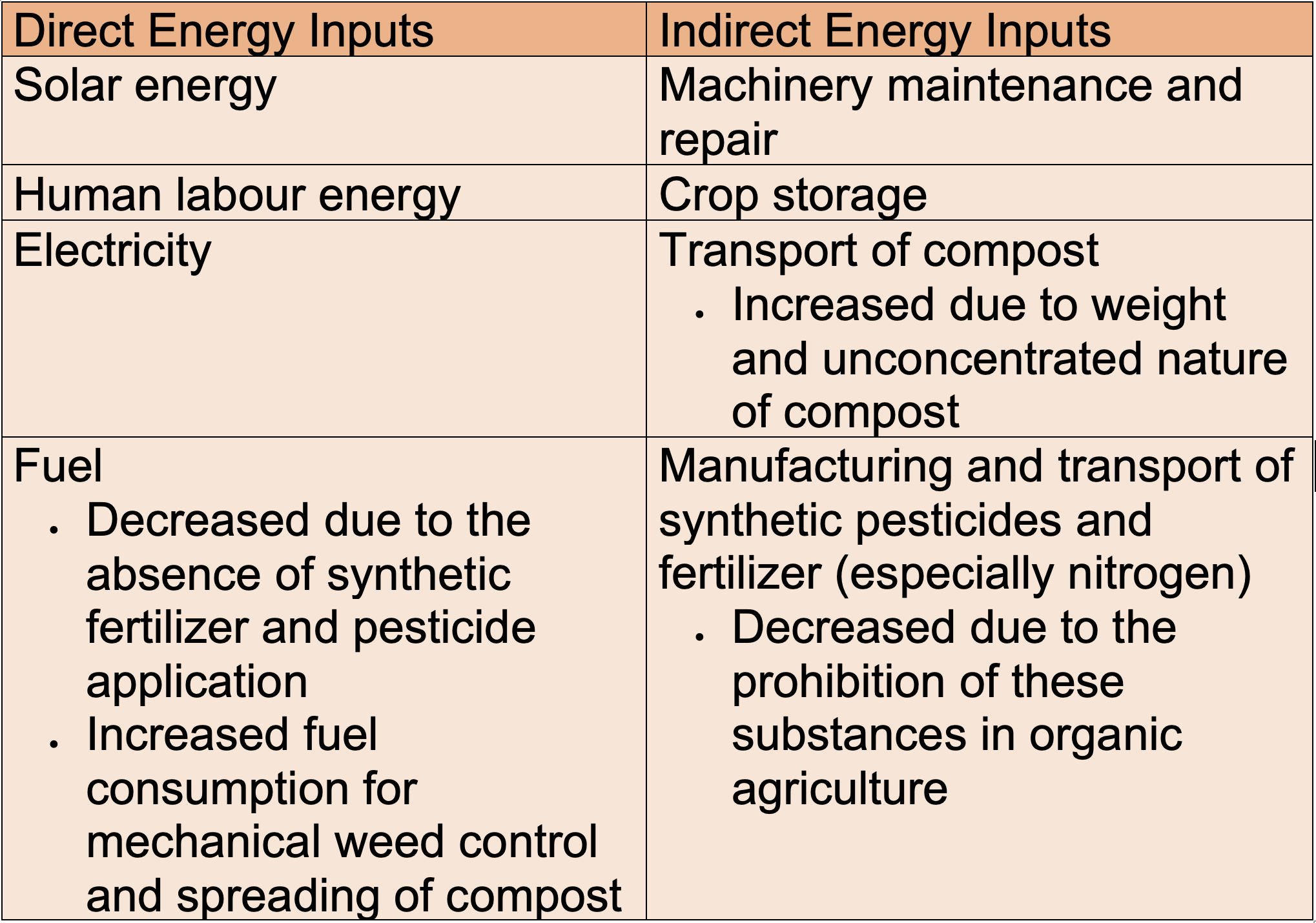
Energy Inputs Comparison: Organics and Conventional Agriculture
The current focus for improving the sustainability of the global food system values strategies that increase yields, while decreasing required inputs. Comparing energy flow in organic and conventional systems is useful for assessing the systems’ respective productivity and has been a topic of debate for over a decade. Numerous factors make the comparison difficult, thereby perpetuating the debate and maintaining confusion for policymakers and the public. These factors will first be explored, then evidence on both sides will be shared, and summarized.
Factors that affect the ability to compare organic and conventional systems in terms of energy input and output are numerous. The most evident issue is the heterogeneous nature of both categories. Organic agriculture is regulated by many different certification bodies so, although there are regulations, they can differ greatly based on the region. Furthermore, in both organic and conventional systems, producers choose a unique set of management strategies based on their preferences and knowledge, the crops they are growing, and their microclimate. This leads to organic production systems that range from just meeting the regional standards, to those that voluntarily incorporate supplemental regenerative and integrative practices that benefit the ecosystem. Conventional systems, that are not regulated even to the point of organics, range from extremely intensive systems with a primary focus on maximizing yields, to systems that resemble some organic systems (Shepherd et al. 2003). Organic agriculture is an established effort to maintain sustainable agriculture; however, this does not mean the current system would solve all agricultural issues if there was a sudden 100% conversion to organic production (Muller et al. 2017).
The existence of regulation, which can change as discoveries surrounding sustainable strategies are made, may help encourage the adoption of sustainable practices as they become clearer through scientific research. The heterogeneous nature of these categories makes individual comparisons of systems difficult to aggregate because results would be different in systems with other crops, farmers, and regions. The energy usage methodologies in studies are also varied which further impedes accurate interstudy comparisons (Paris 2022). Larger scale studies rely on making assumptions when creating averages, which makes results inaccurate when trying to use them in applications other than general recommendations.
Multiple studies have found that organic production leads to a decrease in energy usage per area, and some have also found reduced overall energy usage per unit of food produced. When comparing 13 production methods that represented 90% of sugar beet production in the UK, Tzilivakis et al. (2004) found that organic agricultural systems on fertile soils required less energy input per ton of beets harvested than conventional systems. For cash crop production, a long-term study by Pimentel et al. (2005) at the Rodale Institute compares conventional corn systems that use synthetic nitrogen fertilizer, organic systems that source nitrogen from legume cover crops, and organic systems that rely on animal manure and legumes for nitrogen. The 22-year experiment demonstrated that conventional corn production requires 5.2 million kCal of energy as an input per hectare (Pimentel et al. 2005). The organic legume system required 32% less energy and the organic manure/legume system required 28% less (Pimentel et al. 2005). Yields in this study were found to be lower in the organic systems for the first 5 years, but after the establishment period yields were then similar across the system types in normal weather conditions (Pimentel et al. 2005). However, in drought conditions, both the organic systems produced higher yields than the conventional systems (Pimentel et al. 2005).
A modelling study by Muller et al. (2017) had similar findings – that 100% conversion to organic agriculture would result in a net 19-27% decrease in energy use. This decrease was largely due to the absence of emissions that occur from synthetic fertilizers application, as well as the emissions due to the production of those fertilizers (Muller et al. 2017). The emission reduction may have been under-reported because the study erred on the side of caution when assuming the yield gap between organic and conventional production systems – the average yield gap cited by this study was 8%, but the high side of 25% was used to produce conservative results (Muller et al. 2017). Other emissions that increase with the conversion to organic agriculture include those due to the deforestation that would be required to provide the supplemental agricultural land and slight emission increases from rice and livestock production. The primary conclusion from this study that sets it apart from the Rodale study is that Muller et al. (2017) conclude that organic agriculture conversion is best implemented with two other strategies to create a sustainable food system for a growing population. Reducing human food waste is the first (Muller et al. 2017) and the second is reducing livestock production to decrease the amount of livestock feed being grown on land that would support human food crops (Muller et al. 2017). A reduction in livestock production and an increase in organic agriculture would be complementary, given that an increase in legume production would be required to compensate for the loss of animal protein while also fixing atmospheric nitrogen that would be required to make up for the loss of synthetic nitrogen fertilizer from the previous conventional systems (Muller et al. 2017). A more recent study by Barbieri et al. (2021), seconded this point with the conclusion that a 40 – 60% conversion to organic agriculture could be adopted in conjunction with adjusting the livestock sector and reducing food waste on the demand side of the food supply chain. This conclusion takes the current nitrogen limitations of organics into account (Barbieri et al. 2021).
These studies already highlight that conversion to organic agriculture would be best suited in a situation where other changes to the food system are also made. Other studies have concluded that because organic agriculture does not make a good stand-alone solution, it is inferior to conventional agriculture. Several of these studies make this conclusion due to the yield lags, which they cite are up to 35% rather than 25% (Connor 2018a; Conner 2018b; Kirchmann 2019) and argue that these yield lags would require further deforestation to convert more land for organic (Connor 2018a; Connor 2018b). This is high compared to the recent findings of Alvarez (2021) who reported an average 25% yield gap. More specifically, an average 30% yield gap was found for cereals, and a 10% yield reduction in organic legume production compared to conventional (Alvarez 2021). This study reported that this difference may be due to the inability of cereal crops to sequester nitrogen, compared to the high conventional yields that occur due to the large nitrogen applications at key developmental times (Alvarez 2021).
Studies do not always point out that high applications of nitrogen at specific times may contribute to the higher nitrogen leaching that can occur in conventional production systems (Albarez 2021). Another study, in England and Wales, concluded that the yield lag would lead to an increase in imported food which would increase net energy usage in the food system (Smith et al. 2019). These studies that recommend against the widespread conversion to organic agriculture for a more sustainable agricultural system also focus on nitrogen being limited in organic production systems – legume crop growth and cover crops would not make up for nitrogen gaps left by prohibiting synthetic fertilizers (Kirchmann 2019; Conner 2018b).
Debuschewitz and Sanders (2022) summarize the energy efficiency in the organics vs conventional agriculture debate. Figure 3 below clearly shows that nitrogen limitations are one of the principal unresolved aspects of the debate. The uncertainty caused by normative assumptions, as well as the range of largely unreported reference units also ties in with the aspect of difficulties in interstudy comparisons.
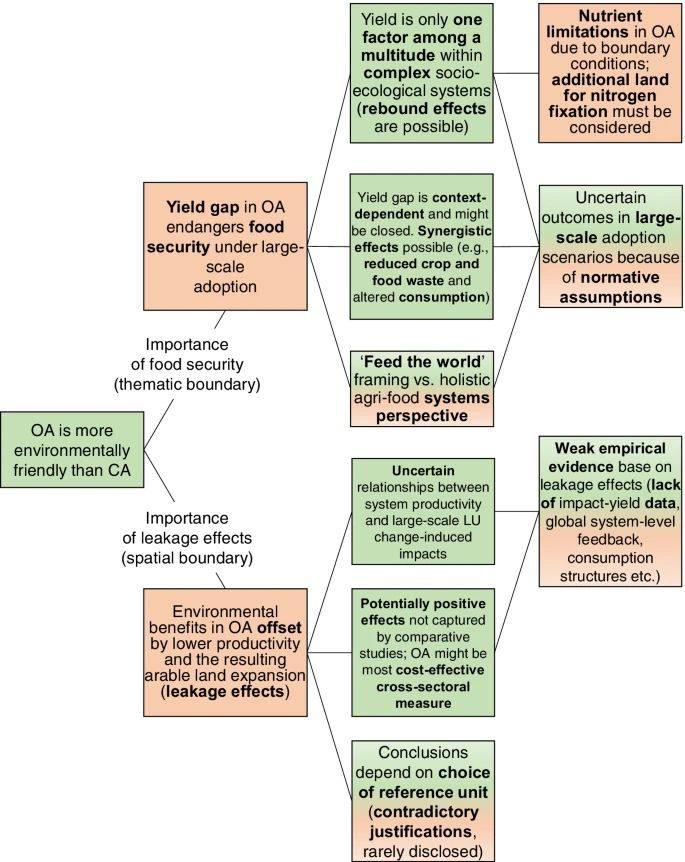
Future of Energy Usage in Organics and the Agricultural System
As this debate continues, the point has been made that our current systems of thinking and study are designed to favour intensive agricultural systems (van der Werf et al. 2020). Life cycle analyses could be improved to better represent less intense production systems, like organic agriculture, by improving measurable indicators of ecosystem services which would aid in better modelling of the indirect effects of agriculture (van der Werf et al. 2020). Reassessing the role of agriculture in society would also be required to better value these less intense systems (van der Werf et al. 2020). Rather than focusing only on the ratio of energy input versus direct energy output (unit of product grown), this ratio could expand to include indirect energy outputs. An example of this would be the energy of providing ecosystem services.
Another important consideration going forward is the evolution of the organic yield gap as a system becomes established. The long-term study performed by Pimentel et al. (2005) found that the yield gap was large during the 5-year establishment period in the two organic systems tested. This is an important point as many studies in organic systems are short-term, and the establishment date of the studied farms is rarely seen as significant information to include when reporting findings. More long-term studies, or studies that include well-established organic systems, may increase our understanding of the yield gap between organic and conventional agriculture.
Conclusion
Energy is an integral part of organic agriculture. Maximizing solar energy usage through increasing photosynthetic productivity is an important focus for improving yields in organic agriculture. Energy efficiency, that is the direct and indirect energy inputs per unit product, is one way to measure the efficiency of current agricultural systems. This method is useful in theory, as current thinking assumes that the best system would have minimal input per unit output; however, the ongoing scientific debate illustrates that a variety of factors make this comparison difficult in reality. Furthermore, it is possible this energy emission ratio should not be the sole measurement of the efficiency of agricultural systems. Creating more concrete indicators of energy savings due to ecosystem services provided by various ecological farming practices might influence how organic agriculture is valued compared to intensive conventional systems.
References
Alvarez R. 2021. Comparing productivity of organic and conventional farming systems: a quantitative review. Arch. Agron. Soil Sci. 28,1-2.
Andrew AC, Higgins CW, Smallman MA, Graham M, Ates S. 2021. Herbage Yield, lamb growth and foraging behavior in agrivoltaic production system. Front. Sustain. Food Syst. 5, 1-12.
Barbieri P, Pellerin S, Seufert V, Smith L, Ramankutty N, Nesme T. 2021. Global option space for organic agriculture is delimited by nitrogen availability. Nature Food. 2(5), 363-72.
Connor DJ. 2018a. Land required for legumes restricts the contribution of organic agriculture to global food security. Outlook Agric. 47(4), 277-82.
Connor DJ. 2018b. Organic agriculture and food security: A decade of unreason finally implodes. Field Crops Res. 225,128-9.
Debuschewitz E, Sanders J. 2022. Environmental impacts of organic agriculture and the controversial scientific debates. Org. Agric. 12(1), 1-15.
Kirchmann H. 2019. Why organic farming is not the way forward. Outlook Agric. 48(1), 22-7.
Muller A, Schader C, El-Hage Scialabba N, Bruggemann J, Isensee A, Erb KH, Smith P, Klocke P, Leiber F, Stolze M, Niggli U. 2017. Strategies for feeding the world more sustainably with organic agriculture. Nature Commun. 8(1), 1-13.
Paris B, Vandorou F, Balafoutis AT, Vaiopoulos K, Kyriakarakos G, Manolakos D, Papadakis G. 2022. Energy use in open-field agriculture in the EU: A critical review recommending energy efficiency measures and renewable energy sources adoption. Renew. Sust. Energ. Rev. 158, 112098.
Pascaris AS, Schelly C, Burnham L, Pearce JM. 2021. Integrating solar energy with agriculture: Industry perspectives on the market, community, and socio-political dimensions of agrivoltaics. Energy Res. Soc. Sci. 75, 102023.
Pascaris AS, Schelly C, Pearce JM. 2020. A first investigation of agriculture sector perspectives on the opportunities and barriers for agrivoltaics. J. Agron. 10(12),1885
Pimentel D, Hepperly P, Hanson J, Douds D, Seidel R. 2005. Environmental, energetic, and economic comparisons of organic and conventional farming systems. J. Biosci. 55(7), 573-582.
Pimentel D. 2009. Reducing energy inputs in the agricultural production system. Mon. 61(3), 92-101.
Shepherd M, Pearce B, Cormack B, Philipps L, Cuttle S, Bhogal A, Costigan P, Unwin R. 2003. An assessment of the environmental impacts of organic farming. Department of Environment, Food and Rural Affairs, UK. DEFRA report: https://www.znrfak.ni.ac.rs/serbian/010-STUDIJE/OAS-3-2/PREDMETI/III%20GODINA/316-KOMUNALNI%20SISTEMI%20I%20ZIVOTNA%20SREDINA/SEMINARSKI%20RADOVI/2013%20OD%20141%20DO%20150%20(1).pdf
Smith LG, Kirk GJD, Jones PJ, Williams AG. 2019. The greenhouse gas impacts of converting food production in England and Wales to organic methods. Nature Commun. 10(1), 1-0.
Tzilivakis J, Warner DJ, May M, Lewis KA, Jaggard K. 2004. An assessment of the energy inputs and greenhouse gas emissions in sugar beet (Beta vulgaris) production in the UK. Agric. Syst. 85, 101-119.
van der Werf HMG, Knudsen MT, Cederberg C. 2020. Towards better representation of organic agriculture in life cycle assessment. Nature Sustain. 3(6), 419-25.