7b: Greenhouse Gases in Organics
Written by Jenna West
Introduction
The management practices common to organic agriculture and their effect on soils and other aspects of agroecosystems present significant opportunities for mitigating greenhouse gas emissions. Carbon dioxide (CO2), nitrous oxide (N2O) and methane (CH4) are the gases commonly associated with agriculture and will be explored in depth in this chapter. How these greenhouse gases are released from soil provides a basis for understanding how the three gases are transformed from other states within the soil. The impact of organic practices on these processes is also highlighted.
Greenhouse Gases
Agricultural greenhouse gases are largely a result of soil outgassing in agroecosystems, except for methane which is largely attributed to livestock. CO2, N2O, and CH4 are problematic in the atmosphere because of their contribution to the greenhouse effect. Similar to the glass of a greenhouse or the windows of a car, these gases allow the short-wave radiation of sunlight to pass through but trap the long-wave radiation that is reflected back toward earth, resulting in warming (Weil and Brady 2016). Each gas has a different level of warming capacity which is called its global warming potential (GWP) (Johnson et al. 2007). CO2 has a GWP of 1, while that of CH4 is about 20, meaning it has 20x more warming potential than CO2 (Government of Canada 2020). N2O is the strongest with a GWP of about 300 (Government of Canada 2020). About 10% of Canada’s greenhouse gas emissions are a result of agricultural activities, not including fossil fuels used for cultivation or fertilizer used in conventional agriculture (Government of Canada 2020). Much of the greenhouse gases produced from agriculture result from management practices that impact agricultural soils (Weil and Brady 2016).
Gas Movement Within Soil
Gases move in soil air spaces, which are present in the soil pores when they are not filled with water (Brown et al. 2021). The amount of air in the soil pores changes with the amount of water, as seen below in Figure 1. Soil air near the surface of the soil profile is similar in composition to that of the atmosphere – with N2, O2, and CO2 present (Brown et al. 2021), while the soil air deeper in the profile is influenced by respiring roots and microorganisms (Weil and Brady 2016; Brown et al. 2021). The availability of oxygen in soil air depends on soil structure, pore connectivity, and soil water content (Weil and Brady 2016). Organic agriculture focuses on the importance of soils because good soil structure promotes aeration which promotes the growth of plants and microbes (King et al. 2020; Brown et al. 2021). When soils are saturated with water or are waterlogged, there is no O2 being added to the soil air, so O2 levels decline as input for root and microorganism respiration (Weil and Brady 2016).
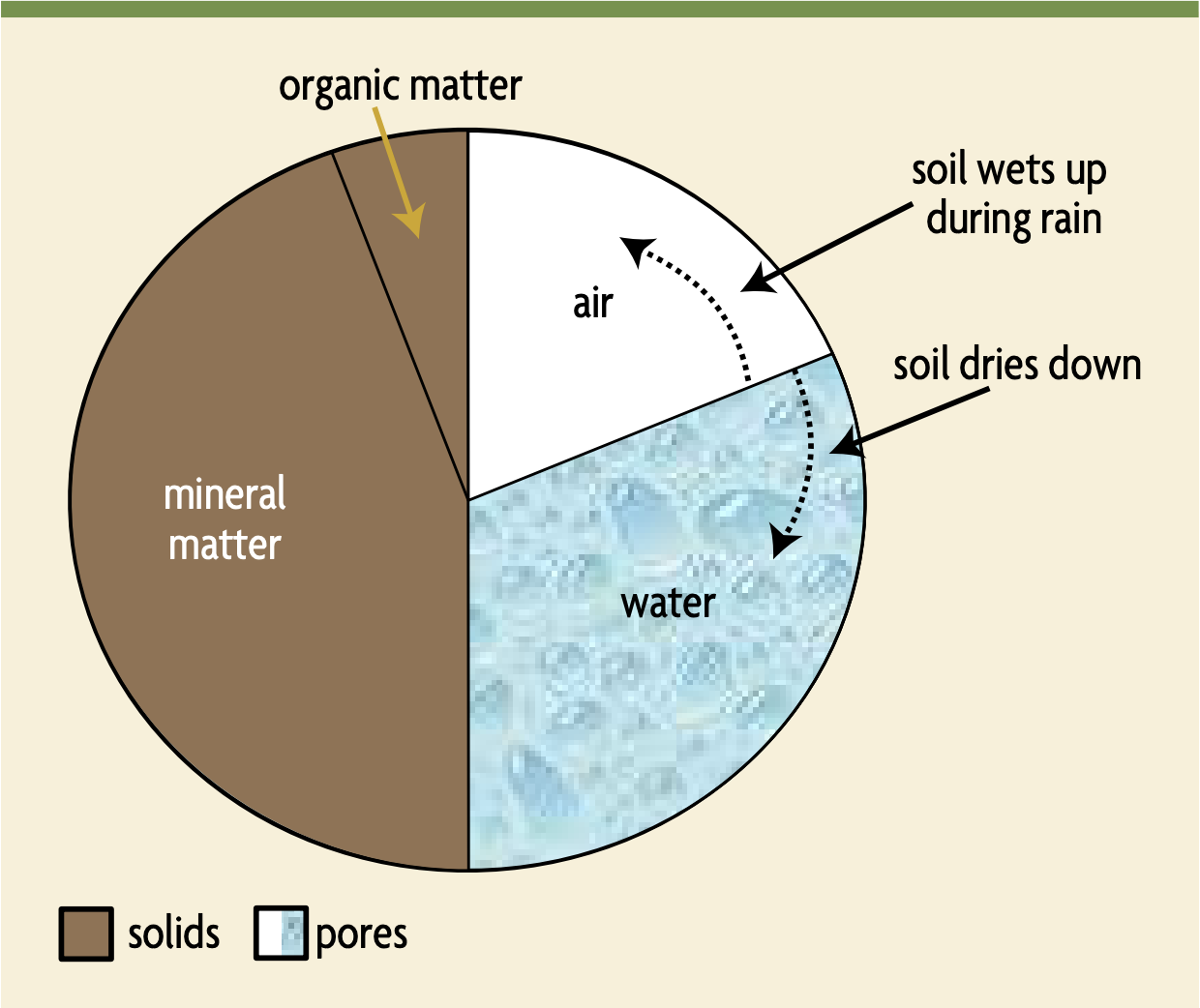
The water-stable aggregates promoted by organic practices, like the addition of nutrients via compost, reduce compaction and enhance aeration and gaseous interchange through the management of soil organic matter (SOM) (King et al. 2020; Tisdall and Oades 1982). Other practices associated with organic agriculture that impact soil organic matter (SOM) will be further explored later in the chapter .
How Nutrients are Transformed
The way nutrients are stored in soils and how they are transformed to gaseous forms that can be released via gaseous interchange provides a base for understanding how greenhouse gases are emitted from soils. As mentioned in Chapter 7c, water-soluble nutrients can dissolve as solutes in soil water, while other nutrients are stored in the soil as insoluble compounds (Munroe 2016). Other nutrients are held on soil surfaces, contained within organic matter, or fixed in clays (Munroe 2016). Greenhouse gases have other forms within the soil, and various mechanisms influenced by agricultural management practices transform them into gaseous forms that act as greenhouse gases when released into the atmosphere.
Nitrogen takes many forms in soil. Nitrogen is plant available as ammonium (NH4+) and nitrate (NO3– ) (Weil and Brady 2016). When nutrients are contained within compost, total nitrogen is a combination of ammonium, nitrate, and organic nitrogen (Clemson University 2022). Nitrogen becomes a problem in the atmosphere in the form of N2O where it acts as a greenhouse gas (Oertel et al. 2016). Levels of N2O production are slow and steady from soils in natural ecosystems like forests but are highly variable in agroecosystems (Weil and Brady 2016). Any periods of waterlogged soil conditions constitute most of the yearly N2O emissions from agroecosystems (Weil and Brady 2016).
N2O is produced in soils in a process called denitrification, shown below in Figure 2 (Weil and Brady; Ussiri and Lal 2012). About 5% of soil microbes are capable of denitrification, including types of bacteria, archaea, and some fungi (Ussiri and Lal 2012). Denitrification occurs because organisms that usually use O2 in respiratory processes do not have access to O2 and nitrate, or NO3–, is the next best option (Ussiri and Lal 2012). This reinforces why applying compost or nitrogen fertilizer before heavy rainfall events, which results in low oxygen levels in soils due to wet conditions, causes higher nitrogen losses than proper application strategies. N2O can be produced in large quantities in wet soils with low oxygen levels (Weil and Brady 2016; Ussiri and Lal 2012). Acidic soil also encourages the production of N2O from the denitrification process. Thus, liming acidic soils can reduce denitrification rates (Ussiri and Lal 2012).

Organic farming systems have been shown to increase N2O emissions. The population of denitrification soil microbes have been found to increase with the application of compost when compared to ammonium nitrate fertilizer which corresponds with higher emissions from organic systems (Dambreville et al. 2006; Charles et al. 2017). Soil carbon is often the limiting factor in denitrification, so when organic amendments increase soil organic carbon (SOC) levels relative to synthetic nitrogen fertilizers, it makes sense this would increase denitrification rates (Charles et al. 2017; Ussiri and Lal 2012). Raw manure leads to the highest rates of N2O emissions. At the same time, combinations of organic and synthetic fertilizers are medium risk, and organic sources like compost and crop residues are low risk for N2O emissions (Charles et al. 2017). No-till has also been seen to increase N2O emissions, for the same reason that it promotes higher levels of SOC (Zhou et al. 2017) and moisture at the same time.
The exact extent of N2O emissions caused by various organic amendments is still uncertain given the variation in composition between different organic sources and the dependence on other soil factors and management practices (Zhou et al. 2017; Charles et al. 2017). For example, N2O emissions are higher in fine-textured soils than coarse ones, perhaps due to N availability (Charles et al. 2017). Well-drained soils, which are also often coarse-textured, promote lower N2O emissions from greater air space and presence of oxygen (Charles et al. 2017). Furthermore, factors such as climate also influence soil N2O emissions as precipitation levels and patterns affect the amount of water present in soil and therefore the presence of anaerobic conditions (Charles et al. 2017). Soils in warm temperate climate zones have been found to facilitate N2O emissions from organically fertilized soils compared to cool temperate climates (Zhou et al. 2017). However, this effect is inconsistent, possibly because the conversion of N2O to N2 is also sometimes stimulated, reducing the amount of N2O that is released in the conversion process (Zhou et al. 2017). Although organic agriculture promotes higher N2O emissions associated with the use of organic amendments, other studies have found that these emissions are offset by the carbon sequestration that occurs in organic systems (Zhou et al. 2017).
Carbon Dioxide (CO2)
Carbon is essential for all life on earth including agricultural systems, and organic practices promote soil organic carbon management (Horwath and Kuzyakov 2018). SOC makes up about half the mass of SOM, or half of all the organic matter in soil (Weil and Brady 2016). Carbon dioxide is produced in the soil as microbes decompose SOM. Carbon flux, the movement of carbon from one pool to another, is highest in the winter and lowest in the summer (Jacinthe et al. 2002). In the summer, crops take in large amounts of CO2 due to high photosynthesis rates, which counters CO2 emissions from soils (Jacinthe et al. 2002).
Organic carbon enters agricultural soils via inputs like plant and root residues and as animal and bio-waste products (Weil and Brady, 2016). Besides organic carbon, inorganic carbon also exists in soils as carbonate, a parent rock material (Bai et al. 2017). Both organic and inorganic carbon act as carbon sinks, or carbon storage, in the agroecosystem as well as in the global carbon cycle (Bai et al. 2017). Within the range of SOC storage, much of the carbon is protected while some is available, ‘labile’ or ‘active’ carbon (Bailey et al. 2019; Weil and Brady 2016).
Agricultural practices have been seen to destabilize passive carbon, increasing levels of active carbon in agricultural soils (Bailey et al. 2019; Weil and Brady 2016). This, in turn, means that agricultural soils generally have lower SOC levels than natural soils (Horwath and Kuzyakov 2018). Tillage is a significant contributor to this as the process physically breaks up soil aggregates, removing the protection that aggregates provide to the carbon they contain (Bailey et al. 2019). Organic agriculture has been found to result in soils with relatively higher SOM and SOC levels than conventional systems (Johnson et al. 2007; Gomiero et al. 2011). Many farm practices, like no-till, cover cropping, mulching, and use of residues, are associated with organic production systems to maximize inputs for increased carbon in soil and/or minimize SOC losses (Weil and Brady 2016). Additionally, adding nutrients via compost and manure is one of the principal reasons organic agriculture has a significant opportunity to sequester carbon (Horwarth and Kuzyakov 2018). Some practices like cover cropping and perennial species between rows in orchards, increase plant biomass production, and therefore the amount of carbon being sequestered via photosynthesis (Horwath and Kuzyakov 2018). Others, like mulching, manure application, and reducing tillage, promote good soil structure which leads to aggregation and the protection of carbon from soil microbes (Weil and Brady 2016).
Carbon sequestration is becoming a more widespread strategy to mitigate rising atmospheric CO2 levels (Horwath and Kuzyakov 2018). As carbon becomes monetized through systems like Canada’s carbon tax, this also becomes an opportunity for farmers to monetize this ecosystem service they are providing via carbon sequestration. Carbon sequestration in this sense is defined as storing carbon long-term to reduce the concentration of atmospheric CO2 (Johnson et al. 2007). Not all the mechanisms surrounding carbon sequestration and carbon destabilization are well understood (Horwath and Kuzyakov 2018). The problem with carbon sequestration is possible limiting factors to the levels of SOC that can be easily sequestered and maintained in agricultural soils (Horwath and Kuzyakov 2018; Tiefenbacher et al. 2021). Very simply, soil texture itself limits SOC (Weil and Brady 2016). The high level of SOC and SOM in sandy soil will be low compared to fine sandy loam soil (Weil and Brady 2016). Nitrogen also plays a role in the amount of SOC a soil can hold because it promotes plant growth which increases the amount of carbon that is sequestered and stabilized during conversion into plant biomass (Weil and Brady 2016). Climate change itself may also impact carbon sequestration, as higher temperatures and the change in precipitation patterns may negatively affect crop productivity and therefore the residues being added to the system (Horwath and Kuzyakov 2018). It is also possible that the active carbon will be decomposed more quickly so the passive carbon will be affected; however, more studies are needed on this subject (Horwath and Kuzyakov 2018).
The priming effect also limits carbon sequestration (Horwath and Kuzyakov 2018). This phenomenon refers to the input of new carbon into the soil resulting in the decomposition of old carbon and SOM (Liu et al. 2020; Tiefenbacher et al. 2021). The mechanism for this process is still not well understood, possibly because it occurs with different microbes in different ecosystems (Liu et al. 2020). The most significant limitation to this might be the phenomenon of carbon saturation (Tiefenbacher et al. 2021). There is a maximum amount of carbon that soil can hold because there is a limited amount of protection by clay and mineral particles and within its aggregates (Tiefenbacher et al. 2021). This suggests that carbon increases could be limited by a maximum range of carbon dependent on the soil texture (Tiefenbacher et al. 2021; Barre et al. 2017). Research is ongoing on this topic to fully understand the limitations of carbon sequestration and how they can be quantified.
Although these limits exist, agriculture will always remove carbon from the system via the food and products being produced from the land, meaning that agricultural soils will always have a carbon deficit to some extent, presenting a continued opportunity for carbon sequestration. Additionally, practices to sequester marginal amounts of carbon implemented in all agroecosystems would have a significant impact on the levels of atmospheric CO2 (Horwath and Kuzyakov 2018). At COP21 UNFCCC in Paris in 2015, this concept became the ‘4PT’ initiative wherein farmers would increase their SOC by 0.4% in the top 40cm of their soil profile each year (Future Policy 2022). This small increase in SOC could have a significant impact on atmospheric carbon levels (Future Policy 2022).
Methane (CH4)
Soils are also a sink for methane – both uptake and release into the atmosphere (Weil and Brady 2016). When soils become anaerobic, microbes produce methane as they decompose SOM (Weil and Brady 2016). This is especially common in rice paddies where saturated conditions are promoted for extended periods of time (Weil and Brady 2016). Termites, which live in some agroecosystems, are also significant producers of methane (Weil and Brady 2016).
Ruminant livestock are the larger concern when it comes to methane emissions from agriculture. Cattle, buffalo, sheep, and goats are the main ruminant livestock that produce methane during their digestion process. Ruminant livestock have been agriculturally important because they are able to digest grasses which humans are unable to digest (Food and Agriculture Organization [FAO] n.d.). Ruminant livestock make grasslands productive that are otherwise not suitable for cropland FAO n.d.). For this reason and because meat is an important part of the diet for many cultures, extensive research has been and is still being done to fully understand the mechanisms of methane production and how management strategies impact it, in order to minimize emissions.
The difference between organic and conventionally raised cattle that impacts greenhouse gas emissions has to do with diet (Buratti et al. 2017). An Italian case study found that organically raised cattle contribute more methane emissions than conventionally raised cattle due to dietary restrictions (Buratti et al. 2017). Organically raised cattle in Italy, as well as in Canada, are required to graze more and have higher levels of forage in their diet than their conventional counterparts (Buratti et al. 2017; Hamilton 2015). As forages are lower in energy compared to the grain that conventional cattle are finished on, they have a longer finishing period and produce more methane during that long period of time (Buratti et al. 2017). Other studies have found that methane emissions from cattle are more dependent on their density on a farm than whether it is organic or conventional (Stalenga and Kawalec 2008) or that grass fed animals have less methane production than concentrated grain feed.
Conclusion
Agriculture presents a problem and an opportunity regarding greenhouse gases in the face of climate change, largely because the choice of management practices affects levels of greenhouse gases that are emitted from agroecosystems. Gas interchange and the cycling of carbon dioxide, nitrous oxide, and methane were explored in this chapter. Soil management plays the primary role in the contribution of agriculture to greenhouse gas emissions, so the organic practices that promote good soil structure naturally influence this. The impact of good soil structure on greenhouse gas production was largely seen to be positive, with some exceptions due to increased microbial activity. As progress is made in monetizing ecosystem services like carbon sequestration and greenhouse gas mitigation, incentives to implement these practices may give rise to the possibility of practices associated with organic agriculture becoming more conventional as perspectives continue to shift.
References
Bai SG, Jiao Y, Yang WZ, Gu P, Yang J, Liu LJ. 2017. Review of progress in soil inorganic carbon research. In IOP Conference Series: Earth and Environmental Science 100 (1), 012129. IOP Publishing.
Bailey VL, Pries CH, Lajtha K. 2019. What do we know about soil carbon destabilization? Environ Res Lett. 14(8), 083004.
Barré P, Angers DA, Basile-Doelsch I, Bispo A, Cécillon L, Chenu C, Chevallier T, Derrien D, Eglin TK, Pellerin S. 2017. Ideas and perspectives: Can we use the soil carbon saturation deficit to quantitatively assess the soil carbon storage potential, or should we explore other strategies? Biogeosciences Discussions. 22, 1-2.
Brown S, Biswas A, Caron J, Dyck M, Si B. 2021. Soil physics. In Krzic M, Walley FL, Diochon A, Paré MC, Farrell RE (Eds.), Digging into Canadian soils: An introduction to soil science. Pinawa, MB: Canadian Society of Soil Science. https://openpress.usask.ca/soilscience/chapter/soil-health-and-management/
Charles A, Rochette P, Whalen JK, Angers DA, Chantigny MH, Bertrand N. 2017. Global nitrous oxide emission factors from agricultural soils after addition of organic amendments: A meta-analysis. Agric Ecosyst Environ. 236, 88-98.
Clemson University. 2022. Compost Interpretation https://www.clemson.edu/public/regulatory/ag-srvc-lab/compost/interpretation.html
Food and Agriculture Organization (FAO). n.d. Reducing enteric methane for improving food security and livelihoods. United Nations. https://www.fao.org/in-action/enteric-methane/background/what-is-enteric-methane/en/
Future Policy. 2022. The 4 per 1000 initiative. https://www.futurepolicy.org/healthy-ecosystems/the-4-per-1000-initiative-soils-for-food-security-and-climate/
Gomiero T, Pimentel D, Paoletti MG. 2011. Environmental impact of different agricultural management practices: conventional vs. organic agriculture. Critical Reviews in Plant Sci. 30(1-2), 95-124.
Government of Canada. 2020. Greenhouse gases and agriculture. https://agriculture.canada.ca/en/agriculture-and-environment/climate-change-and-air-quality/greenhouse-gases-and-agriculture
Hamilton T. 2015. Organic beef production in Ontario. Ontario Ministry of Agriculture, Food, and Rural Affairs. http://www.omafra.gov.on.ca/english/livestock/beef/facts/09-067.htm#2
Horwath WR, Kuzyakov Y. 2018. The potential for soils to mitigate climate change through carbon sequestration. In Developments in Soil Science 35, pp. 61-92. Elsevier.
Jacinthe PA, Lal R, Kimble JM. 2002. Carbon budget and seasonal carbon dioxide emission from a central Ohio Luvisol as influenced by wheat residue amendment. Soil Tillage Res. 67(2), 147-57.
Johnson JM, Franzluebbers AJ, Weyers SL, Reicosky DC. 2007. Agricultural opportunities to mitigate greenhouse gas emissions. Environ Pollut. 150(1), 107-24.
King AE, Ali GA, Gillespie AW, Wagner-Riddle C. 2020. Soil organic matter as catalyst of crop resource capture. Front Environ Sci. 8, 50.
Liu XJ, Finley BK, Mau RL, Schwartz E, Dijkstra P, Bowker MA, Hungate BA. 2020. The soil priming effect: Consistent across ecosystems, elusive mechanisms. Soil Biol Biochem. 140, 107617.
Magdoff F, van Es H. 2021. Building soils for better crops: Ecological management for healthy soils. 4th Edition. College Park (MD). Sustainable Agriculture Research & Education (Program). National Institute of Food and Agriculture (US). 394 p.
Munroe J. 2016. Soil fertility handbook. Publication 611. 3rd Edition. Ontario Ministry of Agriculture, Food and Rural Affairs (OMAFRA). http://www.omafra.gov.on.ca/
Tiefenbacher A, Sandén T, Haslmayr HP, Miloczki J, Wenzel W, Spiegel H. 2021. Optimizing carbon sequestration in croplands: a synthesis. Agronomy 11(5), 882.
Tisdall JM, Oades JM. 1982. Organic matter and water‐stable aggregates in soils. J Soil Sci. 33(2), 141-63.
Ussiri D, Lal R. 2012. Soil emission of nitrous oxide and its mitigation. Springer Science & Business Media.
Weil RR, Brady NC. 2016. The nature of properties of soil. 15th Edition. Global Edition. Harlow (Essex). Pearson Education Limited. 1104 p.
Zhou M, Zhu B, Wang S, Zhu X, Vereecken H, Brüggemann N. 2017. Stimulation of N2O emission by manure application to agricultural soils may largely offset carbon benefits: A global meta‐analysis. Global Change Biol. 23(10), 4068-83.