8a: Predator-Prey dynamics
Written by Taylor Blair
Abstract
This chapter introduces the integral components within the study of predator-prey dynamics in organic agricultural practices. The soil food web is a key food chain in organics and can be divided into interactions between producers, consumers and decomposers. The impact of this web is evident among Nematodes and Mycorrhizal fungi, where nutrient recycling from producers is a top priority. Organic agriculture is directly correlated to an increase in biodiversity due to increased beneficial interactions among plants, pollinators, and natural predators. This being said pests pose a significant risk to organic agriculture as control mechanisms are highly regulated. These pests result in significant decreases in crop yield. A case study of Apiaceae at organic farms ties everything together in examining natural predator-prey dynamics and demonstrates the use of a natural enemy and banker plant systems as effective methods of biocontrol in organics.
Introduction and Impacts
The study of predator-prey interactions in the context of organics has become increasingly necessary due to agricultural intensification and the necessity to fight biodiversity loss in ecological systems (Schmidt et al. 2014). Studies of organic pest communities have uncovered generalized community patterns in response to specific management practices which can, in turn, be used within agricultural landscapes to increase heterogeneity, maintain biodiversity and sustainably increase crop yields (Schmidt et al. 2014).
A predator can be described as an organism that feeds on another organism, whereas the prey is the organism on which the predator feeds (Biology Dictionary 2019). Under the study of organics, the predator is often looked upon as the pest which feeds upon organic produce, which in this context would be denoted as the prey.
The predator-prey dynamic in organic systems and their biological residents must be better understood to better demonstrate their divergent effects. A shift in the natural equilibrium towards a higher population of pests can pose significant harm to many aspects of organic farming. An overwhelming pest presence can be devastating to crop yields and biomass values (Oerke 2006). In contrast, organic farming methods see an increase in biodiversity when compared to conventional systems. Plant species richness is exhibited by enhancing vital functional groups such as plants and pollinators, and amplifying natural pest control (Krauss and Gallenberger 2011).
This chapter will introduce readers to several concepts related to predators’ impacts on the overall health of organic farming. This discussion will begin by exploring major predators and corresponding food chains within biodiverse ecosystems to provide readers with a solid foundation. The chapter will then discuss the role of organic agriculture on biodiversity. A discussion of organic system changes will enable quantification of the effects of pests on biodiversity and how these effects alter organic yield, with the aid of multiple studies and statistical elements. Finally, a case study will be introduced which demonstrates the impact of predator-prey relations on the study of organics.
Major Predators and Food Chains
Within every organic environment, there exists a delicate balance between all of the resident components. Over time, relationships between abiotic and biological components manifest into a complex chain of interactions. This dynamically adjusting balance can be explained in terms of a food chain. From a biological perspective, food chains describe the trophic interactions amongst various groups of organisms involved within a given region (Payne 1980). Concerning organics, the soil food chain demonstrates complex interactions to facilitate nutrient cycling and increase primary productivity rates and carbon storage (Payne 1980).
The soil food web is composed of three central categories that enable all biological interactions in an ecosystem. The first and paramount element to all food chain interactions is the primary consumers. This domain encompasses all elements that rely on carbon and solar energy to facilitate growth, including all plants, lichens, moss, algae and other organic matter. Primary producers are present in every organic farming environment and are critical to the biotic success of other organisms belonging to different trophic levels (USDA n.d.). Producers are the first domain of every food chain and consist mainly of autotrophs that utilize photosynthesis to generate sustenance. Following producers are the consumers, which are herbivores and carnivores that rely on producers’ nourishment as a critical component to their survival. Lastly, the decomposers break down dead plant and animal matter, making nutrients more available to the soil and other parts of ecosystems.
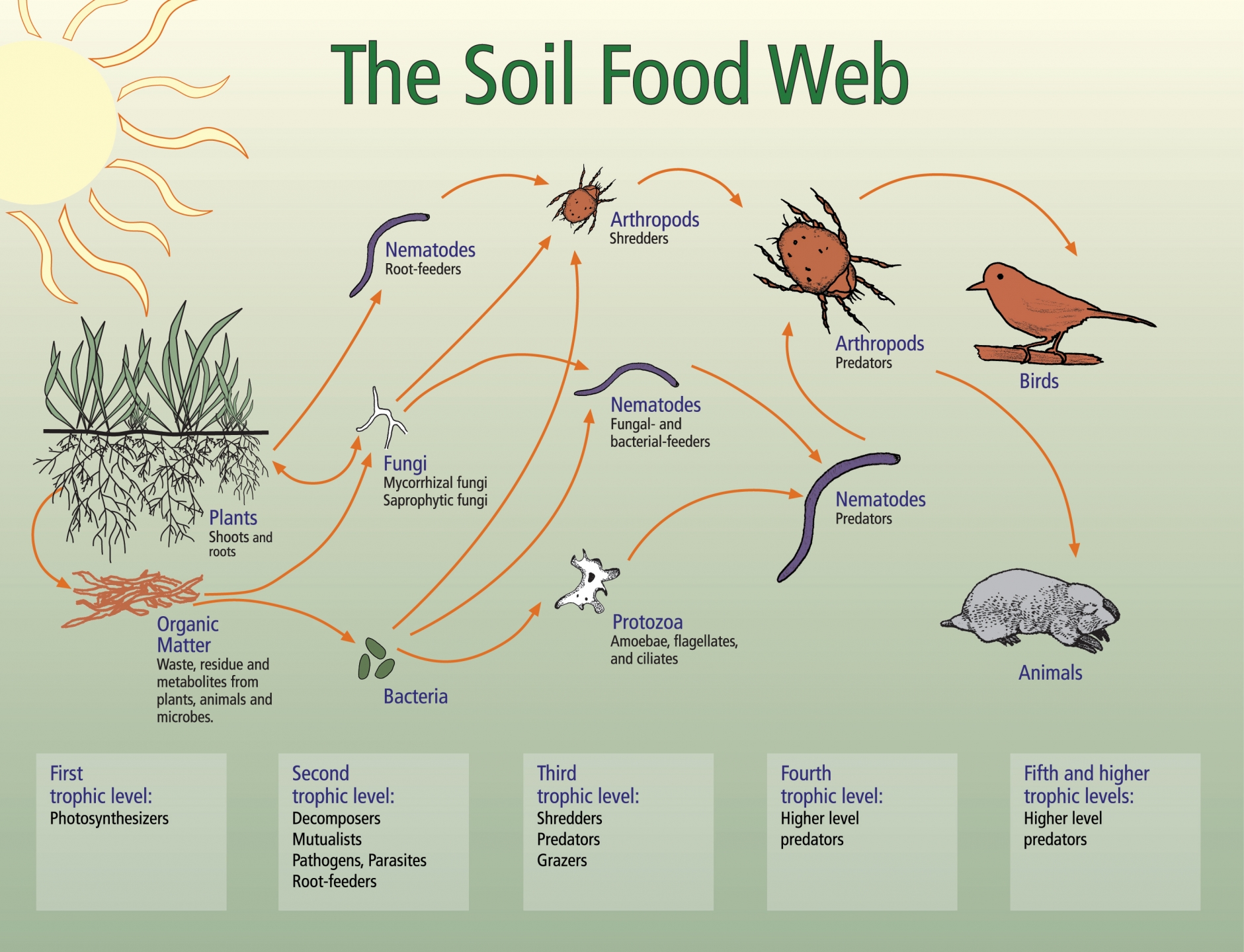
The food webs’ significance lies in their ability to visually and accurately depict the trophic scaffolding that exists within any given ecologically rich region. The structure they provide is of utmost ecological importance to natural communities (Payne 1980). Complex webs can be greatly affected by a loss of any given component, which, in turn, creates a reliance among all involved elements. The chain system works in a cyclical fashion where components at the bottom of the chain share a mutually beneficial relationship with members of different trophic levels (Payne 1980). Subtraction or surplus of one of the categories’ components will have severe consequences on the system’s other parts. For example, scarcity of decomposers in a given region, such as Nematodes or Mycorrhizal fungi, may have substantial impacts on the nutrient cycling and the availability of those nutrients for plant growth (Payne 1980). Without adequate cycling of essential nutrients, this would lead to less production of sustenance and subsequently lower the region’s carrying capacity. This emphasizes that all the components of a given soil food chain are interconnected and reliant upon one another for sustainability and continuing success.
The three central categories of a food chain facilitate the predator-prey relationship amongst the resident species. The prey becomes feed by definition of roles, whereas the predators are the opposing species being fed (Biology Dictionary 2019). This dynamic is often overlooked concerning plants as prey to predators as they appear indifferent to predation pressures (Biology Dictionary 2019). Additionally, plants do not align with the typical view of an exemplary predator-prey relationship. However, there have been many experiments that conclude plants are active participants in the relationship. This can be exemplified through biological defence mechanisms in response to communicated overgrazing (Biology Dictionary 2019). Examples of this in Canadian organics are demonstrated with many apple and tomato varieties. When exposed to overwhelming levels of overgrazing, some species of plants have adapted to release ethylene, a hormone to alert surrounding plant species to the threat (Biology Dictionary 2019). Once received, the plant species will slowly release ethylene gas that will produce toxic substances (Biology Dictionary 2019), resulting in a defence mechanism that is toxic to predators and deters extensive overgrazing.
These complex interactions develop over time and will affect each species’ survival and fitness (Biology Dictionary 2019). Evolution has made it so that predation pressures have facilitated the development of biological defence mechanisms, critical to the success of organics as non-conventional management systems cannot rely on chemical treatments to combat overwhelming pest populations.
Organics and Biodiversity
Biodiversity can be defined as the variety of life amongst all environmental ecosystems. Additionally, biological diversity is a very dynamic component of the natural world affected by many variables and will adjust accordingly (Letourneau and Bothwell 2008). The biodiversity level is heavily dependent on its ecological characteristics, and organic farming environments act as excellent domains (Krauss and Gallenberger 2011). In comparison to conventional agricultural environments, organic systems boast significant increases in biodiversity (Krauss and Gallenberger 2011). A study conducted by Krauss and Gallenberger (2011) on functional diversity in organics versus conventional systems revealed that organic fields possessed five times higher plant species and twenty times higher pollinator species richness when compared to conventional fields. Furthermore, organic farming practices correlate with direct increases in biodiversity and functional groups critical to agriculture’s success (Krauss and Gallenberger 2011).
These functional groups include plants, pollinators, and predators that benefit natural pest control (Krauss and Gallenberger 2011). Additionally, heightened levels of biodiversity in organic systems make them more resilient to any environmental issues. For example, in Canadian agriculture, invasive species pose substantial threats to farming yields from both organic and conventional systems. The introduction of foreign pests can result in catastrophic losses to a region’s biodiversity. However, since organic systems are more ecologically rich than conventional farming systems, they have greater potential to resist and adapt to the imposed environmental change (Letourneau and Bothwell 2008).
These findings demonstrate that holistic management approaches are readily applied in organic practices and contribute to the industry’s success. Holistic practices rely only on natural, ecological cycles including biodiversity to sustain agricultural outputs without conventional pesticides and herbicides (Farag El-Shafie 2020). The benefits of these practices’ to the farmland are heightened soil fertility, increased carbon dioxide storage, reductions in fossil fuel inputs and preservation of biodiversity (Farag El-Shafie 2020). Furthermore, preserving biodiversity facilitated by holistic management practices reduces the primary invasion and the establishment of harmful pests and diseases (Farag El-Shafie 2020).
Effects of Pests on Biodiversity and Yield in Organics
Organic farming is an emerging and successful industry, with the number of farms doubled in the past few years (Shirk et al. 2012). Despite this success, the industry has been struggling to effectively control pest populations within the National Organic Standards (NOS) in the USA. Alternative strategies, including push-pull strategies and banker plants, have become components in integrated pest management for organics yet, despite this, the industry still sees high rates of biodiversity loss and decreased yields (Shirk et al. 2012).
For example, the pest Anasa tristis has been the most challenging pest in organic cucurbits (Schmidt et al. 2014). Many organic farmers refuse to grow cucurbits due to feeding damage and vectored pathogens, which account for greater than 80% of crop yield loss which results in subsequent economic losses for the farm involved (Schmidt et al. 2014). Yield loss comprises two categories: primary and secondary losses (Cerda et al. 2017). Primary losses occur in the specific year that the pest or disease has made an impact, whereas secondary losses are the accumulation of those losses into subsequent years due to the pests and diseases from the previous year (Cerda et al. 2017). In annual crops on organic farms, the accumulation of pests in seeds and soil remaining from the previous year can lead to secondary losses (Cerda et al. 2017). This leads to decreased yield in subsequent years, but soil contamination leads to decreased soil quality (Cerda et al. 2017).
Globally, pests cause a loss in the crop yield of wheat by upwards of 50%, and more than 80% in cotton production (Oerke 2006). 40% of yield loss in maize, rice, and potatoes is due to pests, and these are just a few examples (Oerke 2006). The pests which cause the most significant yield losses are not animal pests or pathogens, which account for losses of 18% and 16%, respectively, but weeds which can cause losses of 34% (Oerke 2006). Weed losses are easily mitigated with conventional farming pesticides, but these losses can be unaddressed in organic farming due to strict regulations (Oerke 2006).
Ultimately, the largest threats posed to organic farming due to predator-prey dynamics are crop yield loss and biodiversity loss. Subsequent economic losses occur at the farmer’s expense due to this dynamic, with few ways to mitigate these challenges due to tight NOS regulations.
Case Study: Arthropod Pest Management in Organic Crops
In Florida, plant species are under constant threat of invasion by predatory pests on organic farms. A study by Shirk et al. (2012) takes a closer look at the dynamic predator-pest relationship on Apiaceae on organic farms, with specific emphasis on flowers of Queen Anne’s lace, Daucus carota L., and false Queen Anne’s lace, Ammi majus L.F. bispinosa (Shirk et al. 2012). With the number of organic farms in Florida doubling recently, the state faces the increasingly significant challenge of providing effective control of predatory flower thrips Frankliniella bispinosa (Thysanoptera: Thripidae) that is compatible with the National Organic Standards (NOS) (Shirk et al. 2012). To control these thrip species within NOS standards, the minute pirate bugs, Orius spp. (Hemiptera: Anthocoridae), which are naturally occurring predators in the Florida region, were introduced (Shirk et al. 2012). The large floral heads of the false Queen Anne’s lace provide an environmental oasis for Orius spp., providing plentiful food and increased mating opportunities that facilitate a sympatric coexistence of the two species in the Florida region (Shirk et al. 2012).
At the end of April, the thrips population’s size experienced a precipitous decline due to the increasing numbers of Orius present (Shirk et al. 2012). The population profile of thrips and Orius spp. on the two flowering plants followed a density-dependent predator response to prey, with a large initial prey population that rapidly declined as the predator population increased (Shirk et al. 2012). Overall, the study demonstrated that the introduction of a natural predator to the plant species provided a cost-effective method of biocontrol, and it is evident that based on the findings, D. carota and A. majus could serve as banker plant systems for Orius spp. (Shirk et al. 2012).
References
Biology Dictionary. 2019. Predator-prey relationship. https://biologydictionary.net/predator-prey-relationship/
Cerda R, Avelino J, Gary C, Tixier P, Lechevallier E, Allinne C. 2017. Primary and secondary yield losses caused by pests and diseases: Assessment and modeling in coffee. PLOS ONE 12(1), e0169133. https://doi.org/10.1371/journal.pone.0169133
Farag El-Shafie HA. 2020. Insect pest management in organic farming system. Dimensions 16, 277-283. DOI: 10.5772/intechopen.84483
Krauss J, Gallenberger I, Steffan-Dewenter I. 2011. Decreased functional diversity and biological pest control in conventional compared to organic crops fields. PLOS ONE 6(5). https://doi.org/10.1371/journal.pone.0019502
Letourneau DK, Bothwell SG. 2008. Comparison of organic and conventional farms: challenging ecologists to make biodiversity functional. Frontiers Ecology Environment 6, 430-438. https://doi.org/10.1890/070081
Oerke E. 2006. Crop losses to pests. Journal Agri. Science 144(1), 31-43. doi:10.1017/S0021859605005708
Payne RT. 1980. Food webs: linkage, interaction, strength and community infrastructure. Journal Animal Ecology, 49(3), 667-685. https://www.jstor.org/stable/i201665
Schmidt JM, Barney SK, Williams MA, Bessin RT, Coolong TW, Harwood JD. 2014. Predator-prey trophic relationships in response to organic management practices. Molecular Ecology 23, 3777-3789. https://doi.org/10.1111/mec.12734
Shirk PD, Shapiro JP, Reitz SR, Thomas JM, Koenig RL, Hay-Roe MM, Buss LJ. 2012. Predator-prey relationships on Apiaceae at an organic farm. Environmental Entomology, 41(3), 487–496, https://doi.org/10.1603/EN11232
United States Department of Agriculture, NRCS. Soil food web. https://www.nrcs.usda.gov/wps/portal/nrcs/detailfull/soils/health/biology/?cid=nrcs142p2_053868