2b: Life in the Soil: The Soil Microbiome
Written by Erin Young & Rylan Carnegie
Introduction
A food web in healthy soil is incredibly diverse, with soils worldwide host to at least a quarter of the world’s living organisms; yet, when biodiversity drops, in both variety and quantity, so does the ability of the soil to recover from disturbance, respond to stresses, and function overall (Tibbett et al. 2020).
Through working with and investing in the soil food web we put it to work for us, as it makes nutrients available for plant support (decreasing the need for the addition of nutrients), defends against pathogens, pests, and disease (reducing the reliance on herbicides, pesticides, etc.), as well as loosening soil and creating pore space, in turn supplying oxygen and carbon dioxide pathways for roots and organisms alike, while at the same time increasing water retention (Lowenfels and Lewis 2010). Increased water retention in healthy soils is another beneficial impact. Most of the microbiome requires moisture to function and survive. Thus, well-functioning food webs help to foster the conditions they thrive upon most (Voroney and Heck 2015).
All soils are different, with a sum of the parts provided by the whole of the local ecosystem. For example, soil from agricultural land, prairies and forest floors differ in microbial communities, pH, organic carbon and nitrogen concentration, mineral content and more (Fierer 2017). The soil in one ecosystem, say, on agricultural land, can have multiple microbial environments and communities mere micrometres apart (Fierer 2017).
A microorganism, or microbe, is an organism not seen by the naked eye. Microorganisms are a part of every domain of life: eukaryotes, bacteria and archaea. In soil, they play a variety of roles, summarized below in Table 1, contributing to soil health and overall environmental health. For example, saprophytic bacteria and fungi decompose organic matter to turn over nutrients (Fortuna 2012). Protozoa and nematodes play roles in transferring nutrients (Fortuna 2012). Metabolic by-products are passed from organism to organism using biogeochemical processes to cycle carbon, nitrogen and water while strengthening the soil structure (Fierer 2017).
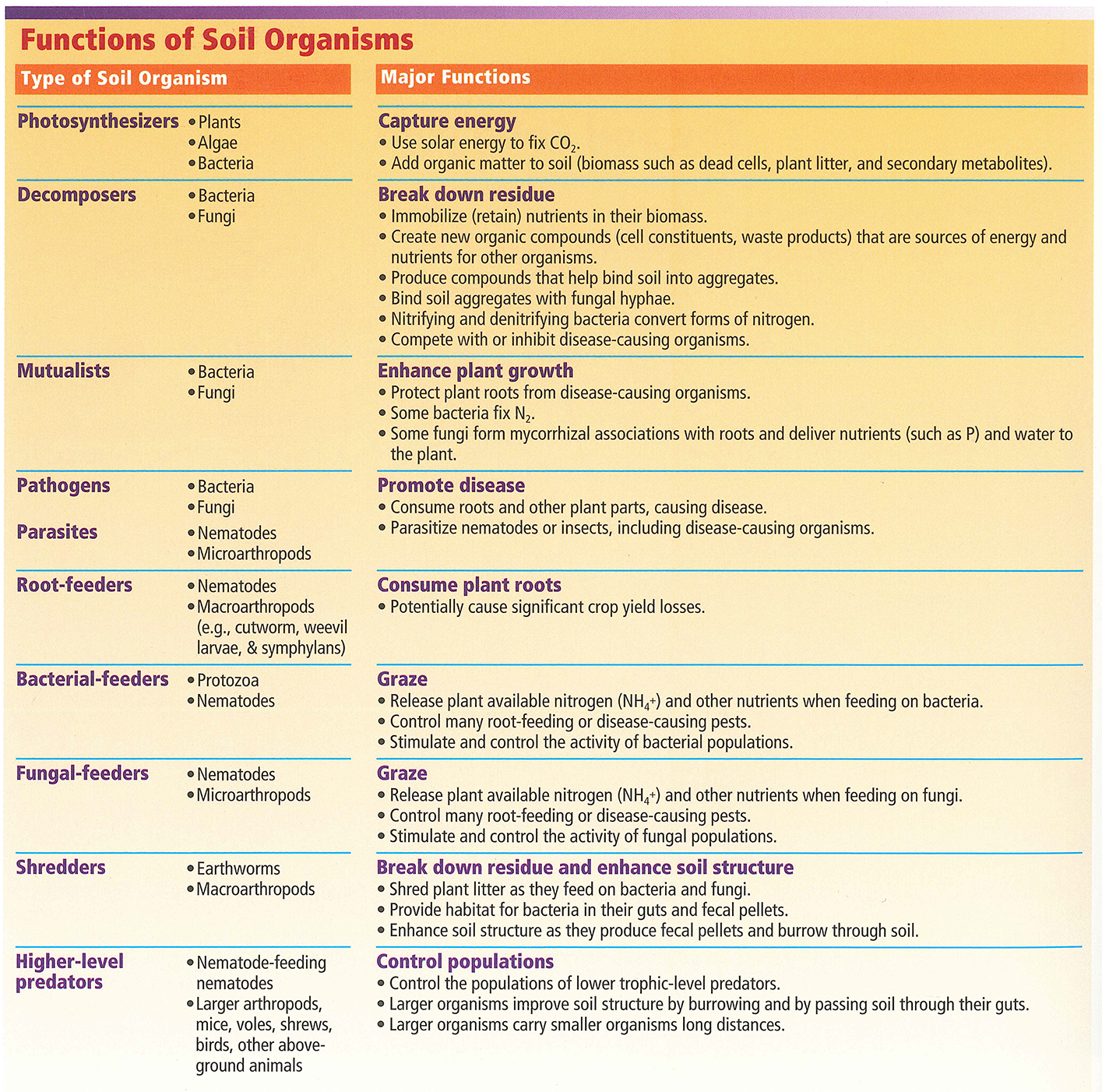
Above-ground and below-ground diversity can also be indicative of soil health (Cardoso et al. 2013). Healthier soil contains a diverse array of above-ground and below-ground species. Greater diversity in the soil correlates with plant resilience and increased plant-beneficial function as the microorganisms work symbiotically, commensally, or neutrally to provide better conditions for the plant to grow (Saleem et al. 2019). Furthermore, a greater overall diversity of microorganisms will create competition between microbes that exploit and compete with plants (Jacoby et al. 2017).
However, less than 1% of soil surface area is occupied by microorganisms, with most microbes remaining dormant due to suboptimal conditions for growth and proliferation (Young and Crawford 2004). In agricultural land, maximizing above-ground productivity and functionality requires optimizing the below-ground environment to encourage microorganism growth (Cardoso et al. 2013). Long-term studies of Organic agriculture practices have demonstrated their capacity to increase species richness in the soil (Lupatini et al. 2017; Hartmann et al. 2014). Conversion to organic farming from conventional farming results in increased presence and activity of various bacteria, fungi and protozoa in the soil (Harkes et al. 2019). It is thus important to investigate the role of soil microbes in each domain of life. Furthermore, organic farming techniques and their impact on soils show that organic practices encourage healthy soils, lessen the environmental impacts of farming, and result in more productive plants.
Eukaryotes
Fungi
The fungal kingdom is composed of single and multicellular eukaryotes that are heterotrophic, meaning they cannot make their food (in contrast to autotrophic plants). Single-celled fungi are known as yeast. The fungal kingdom is distinguished from others by the presence of chitin found in the cell wall. Fungi in the multicellular form have branches of hyphae that make up a network of mycelium.
In soil, fungi are generally very successful organisms due to their ability to change forms to protect themselves from harsh conditions in changing environments. There are three functional groups of fungi, including biological controllers that regulate disease, ecosystem regulators that contribute to soil structure, and decomposers that break down organic matter (Frac et al. 2018).
Furthermore, there are five main phyla of fungi” Chytridiomycota, Zygomycota, Glomeromycota, Ascomycota and Basidiomycota (Aguilar-Marcelino et al. 2020). Chytridiomycota is a type of zoospore-producing fungi that are often obligate and facultative parasites and saprotrophs in the soil (Aguilar-Marcelino et al. 2020). Zygomycota is saprotrophic and these fungi act as decomposers of decaying plant and animal material. Glomeromycota composes half of the known fungi found in soil. This phylum includes the symbiotic mycorrhizal fungi which interact with plant roots to increase the uptake of nutrients in exchange for photosynthates.
This interaction with plant roots makes mycorrhizal fungi especially important in agricultural systems. Organic agriculture aims to build the soil to maximize the services provided by mycorrhizal fungi. Exchanged nutrients include P, Zn, N, Cu and Ca (Smith and Read 2008). There are six groups of mycorrhizal fungi: arbuscular (AM), ectomycorrhizae (EM), monotropoid, arbutoid, orchid, and ericoid (Egerton-Warburton et al. 2005). However, the most common mycorrhizae are in the AM and EM groups. It is worth noting that AM are particularly important because they interact with soil and produce extracellular proteins contributing to water stability (Bedini et al. 2013). Plant roots and mycorrhizal fungi have a symbiotic relationship where plants “trade” exudates in return for water and nutrients. Mycorrhizae access these nutrients with their longer, thinner hyphae (the fungi’s root-like branching network) and effectively increase the area in the soil from which plants are able to use nutrients. It has been noted that greater than 90% of plants are found to form these relationships that can increase their root surface area by 700-1000x (Lowenfels and Lewis 2010). The interacting plants and fungi can also become inter-reliant and dependent on one another for survival (Lowenfels and Lewis 2010).
Fungi play many roles in the soil. They act as plant mutualists, parasites or pathogens, produce metabolites, cycle nutrients, and contribute to soil structure. The presence of different combinations of each of these fungi provides an indicator for soil health, which will be explored in Chapter 6d on One Health. Communities composed of mostly plant pathogens indicate reduced soil quality. Good soil has greater proportions of mycorrhizae, saprotrophs, antagonists, and endophytes (Zhang et al. 2020).
Farming practices have long-term effects on fungal populations and it takes years to develop fungal communities in soil when converting from conventional to organic farming because of prior fungicide use. Fungicides are used to inhibit and kill fungal pathogens to avoid plant disease; however, they also impact beneficial fungi like AM (Chamberlain et al. 2021). Organic agriculture prohibits the use of conventional fungicides but permits the use of organic alternatives. Research indicates that organic fungicides are just as harmful to soil fungi as conventional fungicides (Bereswill et al. 2013). Furthermore, on agricultural land growing soybeans with conventional farming practices, fungal plant pathogens increase in the soil over time (Liu et al. 2019), the use of crop rotation increases the overall fungal biomass, and no-till systems prevent mycorrhizal disturbances (Chamberlain et al. 2021, Chagnon et al. 2013). Intercropping further promotes the diversity of AM, but the application of nitrogen fertilizer decreases AM diversity (Zhang et al. 2020).
Entomopathogenic fungi (EPF) are antagonistic to plant pathogens. Research has found that a higher C:N ratio in the soil contributes to EPF presence, sporulation, growth and virility (Uzman et al. 2019). Organic soils have increased soil carbon due to more carbon sequestration and larger communities of decomposing fungi and research suggests that organic farming, if eliminating fungal inhibitors, as well as integrating no-till, intercropping, and high amounts of nitrogen, will encourage growth and diversity of fungi in the soil (Zhang et al. 2020; Chagnon et al. 2013; Uzman et al. 2019).
Protists (Protozoa)
Protists are largely unicellular eukaryotic organisms that are not plants, animals or fungi (Geisen et al. 2018). Although protists are crucial soil microbes, they cannot be considered soil indicators due to a lack of research (Geisen et al. 2018). Until recently, protists were less studied than fungi, bacteria and higher eukaryotes such as arthropods. Additionally, most protist species are not discovered or described. As scientists are only starting to understand the importance of protists in soil, they remain unable to make significant distinctions regarding how to influence protist diversity.
What scientists have discovered is that protists play significant roles that influence their soil environment. This is evidenced by photoautotrophic protists which are primary producers that make up the bottom of the food web. In peatland soils, protists sequester carbon and contribute to carbon richness (Jassey et al. 2015). Phagotrophic protists consume fungi, bacteria, and other small eukaryotes, thereby regulating and shaping populations (Geisen et al. 2018). Protists that consume microbes also act as mineralizers by freeing the nutrients stored within the bacteria and microbes they consume when they excrete them as waste. Their waste includes carbon and other nutritional compounds, namely ammonium (NH4+), which is then converted into nitrates (if pH is 7 or greater, allowing nitrogen-fixing bacteria to thrive). As much as 80% of plant-required nitrogen comes from the waste of bacteria- and fungi-consuming protozoa. Considering that a large quantity of the bacteria and fungi consumed by protozoa are in the rhizosphere where they are attracted by exudates, these nutrient-rich wastes are delivered right to the roots of plants. As well, these organisms consume organic matter along with their specific prey, which, even if not digested, is still excreted in a more decomposed form (Lowenfels and Lewis, 2010).
Protists may also live as symbionts, acting as parasites, commensals or mutualists with animals, plants, fungi, or even other protists. However, they may be plant pathogens, such as fungi-like oomycotes. Some protists can be predatory and will release nutrients that encourage plant growth. Others are saprotrophic and decompose organic matter (Geisen et al. 2018). The many roles of protists most significantly contribute to nutrient cycling. For example, soil diatoms and euglyphid testate amoebae consume silicon in the soil and contribute to the silicon cycle as much as trees do (Geisen et al. 2018).
Water is a major influence on protists’ environment. A balance of water between drought and excess leads to increased density and diversity of protists since they require water to move. Temperature influences the protist community in soils as well. Flagellate protists favour cooler temperatures (experimentally found to be around 5℃), while amoeboid protist populations favour 23℃ (Opperman et al. 1989). Many other factors impact protist communities, including soil nitrogen, soil carbon, light intensity and plant vegetation.
Arthropods
Arthropods are not microorganisms because they are not microscopic. Instead, arthropods are invertebrates that have segmented bodies and exoskeletons (Coulibaly et al. 2019). Due to their visibility, these arthropods can provide insight into the microbial communities in the soil and serve as an indicator of soil health.
The springtail or Collembola is a hexapod that feeds on fungi, bacteria, and algae (Coulibaly et al. 2019). By eating these other organisms, this hexapod cycles nitrogen and carbon in the soil by moving nutrients and microbes to novel locations, and acts as an indicator of the health of bacterial, fungal, and protozoan communities (Coulibaly et al. 2019; Lowenfels and Lewis 2010). Arthropods like springtails are known as shredders because they break down debris into more readily digestible materials for other organisms, bind together soil particles, and break up hard soils. This work in turn enhances soil fertility, organic matter levels, water-holding capacity and porosity. Multiple studies have shown that Collembola abundance depends on microbial biomass (Filser et al. 2002) which suggests that the practices that harm prokaryotes and lower eukaryotes decrease the prevalence of Collembola.
Some farming practices common to conventional agriculture, such as tillage and pesticide use, negatively impact Collembola communities in the soil (Filser et al. 2002). On the other hand, organic practices like no-till and the addition of compost facilitate microbe abundance and diversity and this may be seen in Collembola and other arthropod populations.
Worms
The presence of worms is evidence of a strong food web, showing that the soil has all the requirements for their survival: fungi, bacteria, organic matter, nematodes, and protozoa. Their waste, known as castings, change the composition of the substrate ingested through biochemical action including microbial decomposition, promoted in part by the bacteria in the gut of the worm which produce digestive enzymes that break the bonds binding nutrients. These digestive enzymes incite the humification process and quick mineralization, which renders nutrients plant available (Ali et al. 2015). Compared to soil that has not been digested by worms, organic matter in castings is bound to seven times more phosphate, five times more nitrogen, three times more plant-available magnesium, ten times more potash, and one and a half times more plant-available calcium (Lowenfels and Lewis, 2010).
Prokaryotes
Bacteria
Bacteria are widely known and understood in soils. They are relevant in the soil microbiome and the wide range of soil bacterial species function in many ways. Bacteria grow and live in water on soil particles and in the rhizosphere surrounding plant roots (Hoorman 2011). These bacteria are aerobic and require oxygen to survive. Anaerobic bacteria are also found in soil but most often are located inside soil particles. In low oxygen or suboptimal conditions for a particular species’ tolerance, some bacteria can go dormant by forming cysts and will remain this way until conditions are again favourable (Segura et al. 2014).
In the carbon cycle, bacteria work with fungi and other microbes to decompose organic carbon and fix carbon into usable forms for plants to use. Bacillus subtilis releases extracellular enzymes to break down hemicellulose in decomposing plant matter (Szmigiel et al. 2021). Pseudomonas fluorescens fix carbon in the soil and are plant growth-promoting bacteria (PGPB) that increase the rates of photosynthesis, intercellular carbon dioxide concentrations and transpiration rates in plants (Wu et al. 2020).
In the nitrogen cycle, free-living and symbiotic nitrogen-fixing bacteria fix otherwise inert nitrogen in the air into usable nitrogen for plants by forming ammonia and hydrogen with the nitrogenase enzyme. These bacteria are extremely beneficial to plants and plant productivity. Rhizobia are symbiotic nitrogen-fixing bacteria that form root nodules on legumes. In these nodules, nitrogen fixation occurs, and the plants are supplied with usable nitrogen, while the bacteria benefit from protection and photosynthates (Bernhard 2010). Rhizobium is an example of plant growth-promoting rhizobium (PGPR) (Bhatti et al. 2017). PGPR also releases plant growth regulators (PGR) in the rhizosphere and promotes plant development. Free-living nitrogen-fixing bacteria include cyanobacteria, among other species. Cyanobacteria form hetero-cyst structures that provide a low-oxygen environment for nitrogenase to function (Bernhard 2010). Nitrogenase is oxygen sensitive, thus, nitrogen-fixing cannot occur in oxygen-rich environments. Other free-living nitrogen-fixing bacteria such as Azotobacter, Pseudomonas and Azospirillum fix nitrogen at night when plants are not photosynthesizing to produce oxygen (Orr et al. 2011).
Nitrification is a process that is performed aerobically by bacteria. There are two steps in nitrification. The first step is ammonia oxidation to nitrite, performed by slow-growing Nitrosomonas, Nitrosopira and Nitrococcus (Bernhard 2010). Archaea also oxidize ammonia (Bernhard 2010). The second step involves the oxidation of nitrite to nitrate. Bacteria that perform nitrite oxidation are slow growing because this process generates little energy. Ammonia and nitrite oxidizers are chemolithotrophic bacteria, meaning they use inorganic compounds as electron donors for energy (Tolli and King 2005). There are many other steps and players in the nitrogen cycle, but these steps and bacteria are the most significant to agriculture.
A significant bacterium in the nitrogen and carbon cycle in the soil is Actinomycetes. These bacteria are free-living nitrogen fixers, but they contribute to many other processes in the rhizosphere of plants (Bhatti et al. 2017). Actinomycetes decompose cellulose, lignin and chitin by hydrolytic enzymes. Resultant nutrients are made available to plants (Bhatti et al. 2017). Additionally, these bacteria degrade pesticides and other inorganic compounds in the soil and can be used for bioremediation (Bhatti et al. 2017). Actinomycetes protect against phytopathogens in the rhizosphere. Given these properties, Actinomycetes are an important indicator of soil health.
Organic agriculture farming practices provide a basis for soil bacteria to flourish. Using compost as fertilizer positively influences the soil microbiome by increasing soil carbon to result in increased crop yield over time (Chocano et al. 2016). Cover crops and no-till similarly benefit the soil microbiome. A long-term study of no-till and cover-crop use showed that cover crops alone lead to higher bacterial numbers but no alterations to the Shannon diversity index (used to estimate species richness and evenness) (Schmidt et al. 2018). The study found that cover crops favoured fast-growing organisms with a range of metabolic capabilities (Schmidt et al. 2018). However, no-till did not alter bacterial density in the soil but it did have a positive effect on the Shannon diversity index. Pairing these practices consequently benefits microbes exponentially.
Archaea
Until recently, archaea were not considered to be the third domain of life. In the 1970s, Carl Woese discovered archaea while studying bacterial ribosomal RNA (rRNA). Woese noted that the structures of rRNA and ribosomal proteins differed between extremophilic organisms and other bacteria (Woese et al. 1990). Archaea visually resemble bacteria because they are unicellular and do not contain membrane-bound organelles like eukaryotes (Londei 2020). However, archaeal genes and molecular structures resemble eukaryotes (Londei 2020).
In soil, archaea play a unique role in the nitrogen cycle. Methanogens are methane-producing organisms. The main influencing factor of archaeal growth in agricultural soil is organic carbon content and the C:N ratio (Karimi et al. 2018). The Shannon diversity index of archaea in soils increases with a greater C:N ratio, as well as with higher amounts of available iron (Jiao et al. 2019). Using organic agriculture farming practices, the C:N ratio of soil increases, supporting the archaea and other soil microorganisms.
Conclusion
The soil in every ecosystem is improved by microbes. Fostering healthy, biodiverse soils is paramount in organic agriculture for increasing yields, plant productivity and nutrient density of crops. Fungi, protists, arthropods, bacteria, and archaea all play diverse and significant roles in the soil microbiome. The health of crops is heavily dependent on the health of the soil, yet it is important to consider the farming practices in play that facilitate or stunt the growth and abundance of microorganisms. Organic agriculture aims to work with and invest in soils by using management practices like no-till, cover-cropping, applying compost, and avoiding the use of pesticides that increase above-ground biodiversity. In this way, organic farmers are able to influence microbes in the soil as well as the soil conditions. Organic practices increase the abundance and diversity of soil microbes which creates competition and resilience against pathogens, pests, and disease. These microbes also increase soil porosity, thereby improving water availability and retention and oxygen availability for plants and soil organisms alike, while also facilitating root growth. Positive effects like increased water retention create a positive feedback loop by fostering a healthy microbiome which thrives due to the increased moisture, among other effects from these practices. By working with the soil and the life it contains, organic agriculture presents an opportunity to produce food while at the same time not reducing soil fertility in the agroecosystems of the future.
References
Aguilar-Marcelino L, Mendoza-de-Gives P, Al-Ani LKT, López-Arellano ME, Gómez-Rodríguez O, Villar-Luna E, Reyes-Guerrero DE. 2020. Using molecular techniques applied to beneficial microorganisms as biotechnological tools for controlling agricultural plant pathogens and pest. Mol. Aspects of Plant Beneficial Microbes in Agric. 333–349
Ali U, Sajid N, Khalid A, Riaz L, Rabbani MM, Syed JH, Malik RN. 2015. A review on vermicomposting of organic wastes. Environ. Progress Sustainable Energy, 34(4), 1050–1062.
Bedini S, Avio L, Sbrana C, Turrini A, Migliorini P, Vazzana C, Giovannetti M. 2013. Mycorrhizal activity and diversity in a long-term organic Mediterranean agroecosystem. Biol. Fertillity Soils 49, 781–790
Bereswill R, Golla B, Streloke M, Schulz R. 2013. Entry and toxicity of organic pesticides and copper in vineyard streams: Erosion rills jeopardize the efficiency of riparian buffer strips. Agric. Ecosyst. Environ. 172, 49–50
Bernhard A. 2010. The Nitrogen Cycle: Processes, Players, and Human Impact. Nature Education, Knowledge project 3(10) https://www.nature.com/scitable/knowledge/library/the-nitrogen-cycle-processes-players-and-human-15644632/
Bhatti AA, Haq S, Bhat RA. 2017. Actinomycetes benefaction role in soil and plant health. Microb. Pathogenesis 111, 458–467
Cardoso EJBN, Vasconcellos RLF, Bini D, Miyauchi MYH, Santos CA dos, Alves PRL, Paula AM de , Nakatani AS, Pereira J de M, Nogueira MA. 2013. Soil health: Looking for suitable indicators. What should be considered to assess the effects of use and management on soil health? Sci. Agric. 70, 274–289
Chagnon PL, Bradley RL, Maherali H, Klironomos JN. 2013. A trait-based framework to understand life history of mycorrhizal fungi. Trends Plant Sci. 18, 484–491
Chamberlain LA, Whitman T, Ané JM, Diallo T, Gaska JM, Lauer JG, Mourtzinis S, Conley SP. 2021. Corn-soybean rotation, tillage, and foliar fungicides: Impacts on yield and soil fungi. Field Crops Res. 262
Chocano C, García C, González D, Melgares de Aguilar J, Hernández T. 2016. Organic plum cultivation in the Mediterranean region: The medium-term effect of five different organic soil management practices on crop production and microbiological soil quality. Agric. Ecosyst. Environ. 221, 60–70
Coulibaly SFM, Winck BR, Akpa-Vinceslas M, Mignot L, Legras M, Forey E, Chauvat M. 2019. Functional assemblages of Collembola determine soil microbial communities and associated functions. Frontiers in Environ. Sci. 7, 1– 12
Egerton-Warburton LM, Johnson NC, Allen EB. 2007. Mycorrhizal community dynamics following nitrogen fertilization: a cross-site test in five grasslands. Ecol. Monographs 77, 527-544.
Fierer N. 2017. Embracing the unknown: Disentangling the complexities of the soil microbiome. Nature Rev. Microbiol. 15, 579–590
Filser J, Mebes KH, Winter K, Lang A, Kampichler C. 2002. Long-term dynamics and interrelationships of soil Collembola and microorganisms in an arable landscape following land use change. Geoderma 105, 201–221
Fortuna A. 2012. The Soil Biota. Nature Education, Knowledge project 3(10) (https://www.nature.com/scitable/knowledge/library/the-soil-biota-84078125)
Frac M, Hannula SE, Belka M, Jȩdryczka M. 2018. Fungal biodiversity and their role in soil health. Front. Microbiol. 9, 1–9
Geisen S, Mitchell EAD, Adl S, Bonkowski M, Dunthorn M, Ekelund F, Fernández LD, Jousset A, Krashevska V, Singer D, Spiegel FW, Walochnik J, Lara E. 2018. Soil protists: A fertile frontier in soil biology research. FEMS Microbiol. Rev. 42, 293–323
Harkes P, Suleiman AKA, Elsen SJJ. Van Den, De Haan JJ, Holterman M, Kuramae EE, Helder J. 2019. Conventional and organic soil management as divergent drivers of resident and active fractions of major soil food web constituents. Sci. Rep. 9, 1–15
Hartmann M, Frey B, Mayer J, Mäder P, Widmer F. 2015. Distinct soil microbial diversity under long-term organic and conventional farming. Nature ISME 9, 1177–1194
Hoorman JJ. 2011. The Role of Soil Bacteria. Ohio State Univ. Ext. 1–4 https://ohioline.osu.edu/factsheet/anr-36
Jacoby R, Peukert M, Succurro A, Koprivova A, Kopriva S. 2017. The role of soil microorganisms in plant mineral nutrition—current knowledge and future directions. Frontiers Plant Sci. 8, 1–19
Jassey VEJ, Signarbieux C, Hättenschwiler S, Bragazza L, Buttler A, Delarue F, Fournier B, Gilbert D, Laggoun-Défarge F, Lara E, Mills RTE, Mitchell EAD, Payne RJ, Robroek BJM. 2015. An unexpected role for mixotrophs in the response of peatland carbon cycling to climate warming. Sci. Rep. 5, 1–10
Jiao S, Xu Y, Zhang J, Lu Y. 2019. Environmental filtering drives distinct continental atlases of soil archaea between dryland and wetland agricultural ecosystems. Microbiome 7, 1–13
Karimi B, Terrat S, Dequiedt S, Saby NPA, Horrigue W, Lelièvre M, Nowak V, Jolivet C, Arrouays D, Wincker P, Cruaud C, Bispo A, Maron PA, Bouré NCP, Ranjard L. 2018. Biogeography of soil bacteria and archaea across France. Sci. Adv. 4
Liu H, Pan F, Han X, Song F, Zhang Z, Yan J, Xu Y. 2019. Response of soil fungal community structure to long-term continuous soybean cropping. Frontiers Microbiol. 10, 1–13
Londei P. 2020. Archaeal Ribosomes. Encyclopedia of Life Science 1–5. https://doi.org/10.1002/9780470015902.a0000293.pub3
Lowenfels J, Lewis W. 2010. Teaming with Microbes – A Gardener’s Guide to the Soil Food Web (Revised). Timber Press, Inc.
Lupatini M, Korthals GW, de Hollander M, Janssens TKS, Kuramae EE. 2017. Soil microbiome is more heterogeneous in organic than in conventional farming system. Frontiers Microbiol. 7, 1–13
Opperman MH, Wood M, Harris PJ. 1989. Changes in microbial populations following the application of cattle slurry to soil at two temperatures. Soil Biol. Biochem. 21(2), 263-268
Orr CH, James A, Leifert C, Cooper JM, Cummings SP. 2011. Diversity and activity of free-living nitrogen-fixing bacteria and total bacteria in organic and conventionally managed soils. Appl. Environ. Microbiol. 77, 911–919
Saleem M, Hu J, Jousset A. 2019. More Than the Sum of Its Parts: Microbiome Biodiversity as a Driver of Plant Growth and Soil Health. Annu. Rev. Ecol. Evol. Syst. 50, 145–168
Schmidt R, Gravuer K, Bossange AV, Mitchell J, Scow K. 2018. Long-term use of cover crops and no-till shift soil microbial community life strategies in agricultural soil. PLoS One 13, 1–19
Segura D, Núñez C, Espín G. 2014. Azotobacter Cysts. Encyclopedia of Life Sciences 1–8
Smith S, Read D. 2008. Mycorrhizal Symbiosis. 3rd Edition, Academic Press, London.
Szmigiel I, Kwiatkowska D, Łukaszewicz M, Krasowska A. 2021. Xylan decomposition in plant cell walls as an inducer of surfactin synthesis by Bacillus subtilis. Biomolecules 11, 1–14
Tibbett M, Fraser TD, Duddigan S. 2020. Identifying potential threats to soil biodiversity. PeerJ, 8, e9271. https://doi.org/10.7717/peerj.9271
Tolli J, King GM. 2005. Diversity and structure of bacterial chemolithotrophic communities in pine forest and agroecosystem soils. Appl. Environ. Microbiol. 71, 8411–8418
USDA-NRCS, & Ingham, E. R. The Soil Food Web; Functions of Soil Organisms. Retrieved February 18, 2021. https://www.nrcs.usda.gov/wps/portal/nrcs/detailfull/soils/health/biology/?cid=nrcs142p2_053868
Uzman D, Pliester J, Leyer I, Entling MH, Reineke A. 2019. Drivers of entomopathogenic fungi presence in organic and conventional vineyard soils. Appl. Soil Ecol. 133, 89–97
Voroney RP, Heck RJ. 2015. The Soil Habitat. Chapter 2 In E. A. Paul (Ed.), Soil Microbiology Ecology and Biochemistry (4th ed.). Elsevier Inc. https://doi.org/10.1016/B978-0-12-415955-6.00002-5
Woese CR, Kandler O, Wheelis ML. 1990. Towards a natural system of organisms: Proposal for the domains Archaea, Bacteria, and Eukarya. PNAS. 87, 4576–4579
Wu Y, Ma L, Liu Q, Sikder Md M, Vestergård M, Zhou K, Wang Q, Yang X, Feng Y. 2020. Pseudomonas fluorescence promote photosynthesis, carbon fixation and cadmium phytoremediation of hyperaccumulator Sedum alfredii. Sci. Total Environ. 726
Young, I, Crawford J. 2004. Interactions and Self-Organization in the Soil-Microbe complex. Science 304(5677), 1634-1637.
Zhang R, Mu Y, Li X, Li S, Sang P, Wang X, Wu H, Xu N. 2020. Response of the arbuscular mycorrhizal fungi diversity and community in maize and soybean rhizosphere soil and roots to intercropping systems with different nitrogen application rates. Sci. Total Environ. 740