Dairy Processing
23 Homogenization of Milk and Milk Products
The following topics will be covered in this section:
- Introduction
- Homogenization Mechanisms
- turbulence
- cavitation
- Effect Of Homogenization
- fat globule properties
- surface layers
Introduction
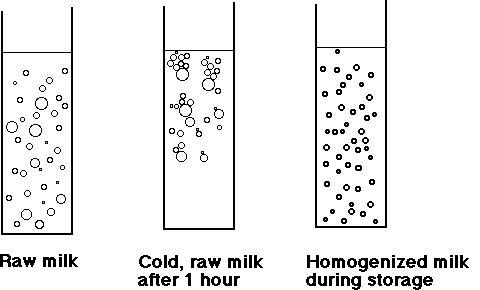
Homogenization Mechanism
To understand the mechanism, consider a conventional homogenizing valve processing an emulsion such as milk at a flow rate of 20,000 l/hr. at 14 MPa (2100 psig). As it first enters the valve, liquid velocity is about 4 to 6 m/s. It then moves into the gap (see below) between the valve and the valve seat and its velocity is increased to 120 meter/sec in about 0.2 millisec. The liquid then moves across the face of the valve seat (the land) and exits in about 50 microsec. The homogenization phenomena is completed before the fluid leaves the area between the valve and the seat, and therefore emulsification is initiated and completed in less than 50 microsec. The whole process occurs between 2 pieces of steel in a steel valve assembly. The product may then pass through a second stage valve similar to the first stage. While most of the fat globule reduction takes place in the first stage, there is a tendency for clumping or clustering of the reduced fat globules. The second stage valve permits the separation of those clusters into individual fat globules.
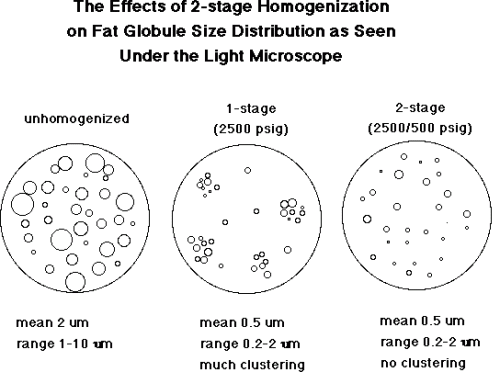
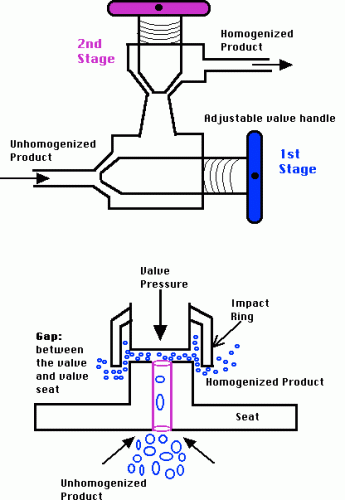
It is most likely that a combination of two theories, turbulence and cavitation, explains the reduction in size of the fat globules during the homogenization process.
Turbulence
Energy, dissipating in the liquid going through the homogenizer valve, generates intense turbulent eddies of the same size as the average globule diameter. Globules are thus torn apart by these eddy currents reducing their average size.
Cavitation
Considerable pressure drop with change of velocity of fluid. Liquid cavitates because its vapor pressure is attained. Cavitation generates further eddies that would produce disruption of the fat globules. The high velocity gives liquid a high kinetic energy which is disrupted in a very short period of time. Increased pressure increases velocity. Dissipation of this energy leads to a high energy density (energy per volume and time). Resulting diameter is a function of energy density.
In summary, the homogenization variables are:
- type of valve
- pressure
- single or two-stage
- fat content
- surfactant type and content
- viscosity
- temperature
Also to be considered are the droplet diameter (the smaller, the more difficult to disrupt), and the log diameter which decreases linearly with log P and levels off at high pressures.
Effect of Homogenization
No Homogenization | 15 MPa (2500 psig) | |
---|---|---|
Av. diam. (µ m) | 3.3 | 0.4 |
Max. diam. (µ m) | 10 | 2 |
Surf. area (m2/ml of milk) | 0.08 | 0.75 |
Number of globules (µ m-3) | 0.02 | 12 |
Surface layers The milk fat globule has a native membrane, picked up at the time of secretion, made of amphiphilic molecules with both hydrophilic and hydrophobic sections. This membrane lowers the interfacial tension resulting in a more stable emulsion. During homogenization, there is a tremendous increase in surface area and the native milk fat globule membrane (MFGM) is lost. However, there are many amphiphilic molecules present from the milk plasma that readily adsorb: casein micelles (partly spread) and whey proteins. The interfacial tension of raw milk is 1-2 mN/m, immediately after homogenization it is unstable at 15 mN/m, and shortly becomes stable (3-4 mN/m) as a result of the adsorption of protein. The transport of proteins is not by diffusion but mainly by convection. Rapid coverage is achieved in less than 10 sec but is subject to some rearrangement.
Surface excess is a measure of how much protein is adsorbed; for example 10 mg/m2 translates to a thickness of adsorbed layer of approximately 15 nm.