Dairy Chemistry and Physics
9 Milk Proteins- Caseins; Casein Micelles; Whey Proteins; Enzymes
Milk Proteins
Milk Protein Fractionation
The nitrogen content of milk is distributed among caseins (75%), whey proteins (18%), miscellaneous proteins (2%) and non-protein nitrogen (5%). This nitrogen distribution can be determined by the Rowland fractionation method:
- Precipitation at pH 4.6 – separates caseins from whey nitrogen
- Precipitation with sodium acetate and acetic acid (pH 5.0) – separates total proteins from whey NPN
Ninety-five percent of the nitrogen is associated with protein. The average concentration of proteins in milk is as follows (although there can be considerable natural variation):
grams/ litre | % of total protein | |
---|---|---|
Total Protein | 33.0 | 100 |
Total Caseins | 26.0 | 78.8 |
alpha s1-casein | 10.7 | 32.4 |
alpha s2-casein | 2.8 | 8.5 |
beta-casein | 8.6 | 26.1 |
kappa-casein | 3.1 | 9.4 |
gamma-casein | 0.8 | 2.4 |
Total Whey Proteins | 6.4 | 19.4 |
alpha lactalbumin | 1.2 | 3.6 |
beta lactoglobulin | 3.2 | 9.8 |
BSA | 0.4 | 1.2 |
Immunoglobulins | 0.8 | 2.4 |
Proteose peptone | 0.8 | 2.4 |
Miscellaneous | 0.6 | 1.8 |
Caseins, as well as their structural form- casein micelles, whey proteins, and milk enzymes will now be examined in further detail.
Caseins
The conformation of caseins is much like that of denatured globular proteins. The high number of proline residues in caseins causes particular bending of the protein chain and inhibits the formation of close-packed, ordered secondary structures. Caseins contain no disulfide bonds. As well, the lack of tertiary structure accounts for the stability of caseins against heat denaturation because there is very little structure to unfold. Without a tertiary structure there is considerable exposure of hydrophobic residues. This results in strong association reactions of the caseins and renders them insoluble in water.
Within the group of caseins, there are several distinguishing features based on their charge distribution and sensitivity to calcium precipitation:
alpha(s1)-casein: (molecular weight 23,000; 199 residues, 17 proline residues)
Two hydrophobic regions, containing all the proline residues, separated by a polar region, which contains all but one of eight phosphate groups. It can be precipitated at very low levels of calcium.
alpha(s2)-casein: (molecular weight 25,000; 207 residues, 10 prolines)
Concentrated negative charges near N-terminus and positive charges near C-terminus. It can also be precipitated at very low levels of calcium.
ß -casein: (molecular weight 24,000; 209 residues, 35 prolines)
Highly charged N-terminal region and a hydrophobic C-terminal region. Very amphiphilic protein acts like a detergent molecule. Self association is temperature dependant; will form a large polymer at 20° C but not at 4° C. Less sensitive to calcium precipitation.
kappa-casein: (molecular weight 19,000; 169 residues, 20 prolines)
Very resistant to calcium precipitation, stabilizing other caseins. Rennet cleavage at the Phe105-Met106 bond eliminates the stabilizing ability, leaving a hydrophobic portion, para-kappa-casein, and a hydrophilic portion called kappa-casein glycomacropeptide (GMP), or more accurately, caseinomacropeptide (CMP).
Structure: The Casein Micelle
Casein micelle image from Dalgleish, D. G., P. Spagnuolo and H. D. Goff. 2004. A possible structure of the casein micelle based on high-resolution field-emission scanning electron microscopy. International Dairy Journal. 14: 1025-1031. This micelle is 120 nm in diameter.
There have been many models developed over the years to explain the structure of the casein micelle, based on all of the information available about its composition and reactivity. The casein sub-micelle model was prominent for many years, but there is sufficient evidence now to conclude that there is not a defined sub-micellar structure to the micelle at all. More recent models suggest a more open structure comprised of aggregates of protein around calcium phosphate nanoclusters. Each of the casein proteins has unique abilitites to either bind with CaP or with other caseins, which gie rise to the aggregates. The nanoclusters provide regions of more or less density. The structure is sufficiently porous to hold a considrable amount of water, and for the surface, and even part of the interior, to be reactive to other substances. All models agree that the k-casein is mostly present as a stabilizng layer around the exterior of the micelle. Please see any of the following references for great detail about micelle structures and models.
Casein Micelle Stability
- the free casein molecules and submicelles
- the free submicelles and micelles
- the dissolved colloidal calcium and phosphate
The following factors must be considered when assessing the stability of the casein micelle:
Role of Ca++:
More than 90% of the calcium content of skim milk is associated in some way or another with the casein micelle. The removal of Ca++ leads to reversible dissociation of ß -casein without micellular disintegration. The addition of Ca++ leads to aggregation.
H Bonding:
Some occurs between the individual caseins in the micelle but not much because there is no secondary structure in casein proteins.
Disulfide Bonds:
alpha(s1) and ß-caseins do not have any cysteine residues. If any S-S bonds occur within the micelle, they are not the driving force for stabilization.
Hydrophobic Interactions:
Caseins are among the most hydrophobic proteins and there is some evidence to suggest they play a role in the stability of the micelle. It must be remembered that hydrophobic interactions are very temperature sensitive.
Electrostatic Interactions:
Some of the subunit interactions may be the result of ionic bonding, but the overall micellar structure is very loose and open.
van der Waals Forces:
No success in relating these forces to micellular stability.
Steric stabilization:
As already noted, the hairy layer interferes with interparticle approach.
There are several factors that will affect the stability of the casein micelle system:
Salt content:
affects the calcium activity in the serum and calcium phosphate content of the micelles.
pH:
lowering the pH leads to dissolution of calcium phosphate until, at the isoelectric point (pH 4.6), all phosphate is dissolved and the caseins precipitate.
Temperature:
at 4° C, beta-casein begins to dissociate from the micelle, at 0° C, there is no micellar aggregation; freezing produces a precipitate called cryo-casein.
Heat Treatment:
whey proteins become adsorbed, altering the behaviour of the micelle.
Dehydration:
by ethanol, for example, leads to aggregation of the micelles.
When two or more of these factors are applied together, the effect can also be additive.
Casein Micelle Aggregation
1. Enzymatic – chymosin (rennet) or other proteolytic enzymes as in Cheese manufacturing.
Chymosin, or rennet, is most often used for enzyme coagulation. During the primary stage, rennet cleaves the Phe(105)-Met(106) linkage of kappa-casein resulting in the formation of the soluble CMP which diffuses away from the micelle and para-kappa-casein, a distinctly hydrophobic peptide that remains on the micelle. The patch or reactive site, as illustrated in the image below, that is left on the micelles after enzymatic cleavage is necessary before aggregation of the paracasein micelles can begin.
During the secondary stage, the micelles aggregate, as illustrated on the right below. This is due to the loss of steric repulsion of the kappa-casein as well as the loss of electrostatic repulsion due to the decrease in pH. As the pH approaches its isoelectric point (pH 4.6), the caseins aggregate. The casein micelles also have a strong tendency to aggregate because of hydrophobic interactions. Calcium assists coagulation by creating isoelectric conditions and by acting as a bridge between micelles. The temperature at the time of coagulation is very important to both the primary and secondary stages. With an increase in temperature up to 40° C, the rate of the rennet reaction increases. During the secondary stage, increased temperatures increase the hydrophobic reaction. The tertiary stage of coagulation involves the rearrangement of micelles after a gel has formed. There is a loss of paracasein identity as the milk curd firms and syneresis begins.
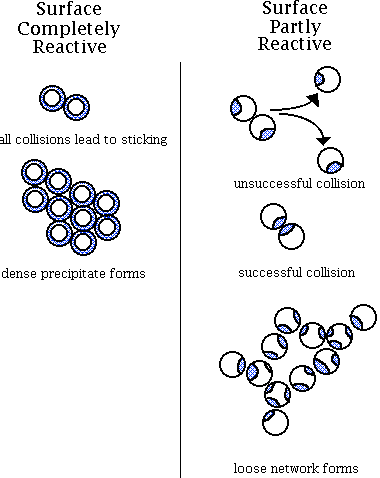
2. Acid. Acidification causes the casein micelles to destabilize or aggregate by decreasing their electric charge to that of the isoelectric point. At the same time, the acidity of the medium increases the solubility of minerals so that organic calcium and phosphorus contained in the micelle gradually become soluble in the aqueous phase. Casein micelles disintegrate and casein precipitates. Aggregation occurs as a result of entropically driven hydrophobic interactions.
3. Heat. Milk is generally very stable to heat up to 90-95oC. At temperatures above the boiling point casein micelles will irreversibly aggregate. On heating, the buffer capacity of milk salts change, carbon dioxide is released, organic acids are produced, and tricalcium phophate and casein phosphate may be precipitated with the release of hydrogen ions.
4. Age gelation. Age gelation is an aggregation phenomenon that affects shelf-stable, sterilized dairy products, such as concentrated milk and UHT milk products. After weeks to months storage of these products, there is a sudden sharp increase in viscosity accompanied by visible gelation and irreversible aggregation of the micelles into long chains forming a three-dimensional network. The actual cause and mechanism is not yet clear, however, some theories exist:
- Proteolytic breakdown of the casein: bacterial or native plasmin enzymes that are resistant to heat treatment may lead to the formation of a slow gel forming over a long period of time.
- Chemical reactions: polymerization of casein and whey proteins due to Maillard type or other chemical reactions
- Formation of kappa-casein-ß -lactoglobulin complexes
An excellent source of information on casein micelle stability can be found in Walstra et al., 2006.
Whey Proteins
ß -Lactoglobulins: (MW – 18,000; 162 residues) This group, including eight genetic variants, comprises approximately half the total whey proteins. ß -Lactoglobulin has two internal disulfide bonds and one free thiol group. The conformation includes considerable secondary structure and exists naturally as a noncovalent linked dimer. At the isoelectric point (pH 3.5 to 5.2), the dimers are further associated to octamers but at pH below 3.4, they are dissociated to monomers.
alpha-Lactalbumins: (MW – 14,000; 123 residues) These proteins contain eight cysteine groups, all involved in internal disulfide bonds, and four tryptophan residues. alpha-Lactalbumin has a highly ordered secondary structure, and a compact, spherical tertiary structure. Thermal denaturation and pH <4.0 results in the release of bound calcium.
Enzymes
- lipoprotein lipase
- plasmin
- alkaline phosphatase
Lipoprotein lipase (LPL): A lipase enzyme splits fats into glycerol and free fatty acids. This enzyme is found mainly in the plasma in association with casein micelles. The milkfat is protected from its action by the FGM. If the FGM has been damaged, or if certain cofactors (blood serum lipoproteins) are present, the LPL is able to attack the lipoproteins of the FGM. Lipolysis may be caused in this way.
Plasmin: Plasmin is a proteolytic enzyme; it splits proteins. Plasmin attacks both ß -casein and alpha(s2)-casein. It is very heat stable and responsible for the development of bitterness in pasteurized milk and UHT processed milk. It may also play a role in the ripening and flavour development of certain cheeses, such as Swiss cheese.
Alkaline phosphatase: Phosphatase enzymes are able to split specific phosporic acid esters into phosphoric acid and the related alcohols. Unlike most milk enzymes, it has a pH and temperature optima differing from physiological values; pH of 9.8. The enzyme is destroyed by minimum pasteurization temperatures, therefore, a phosphatase test can be done to ensure proper pasteurization.