Hypothalamus and Pituitary
As discussed in the previous chapters the endocrine system is a network of glands that secrete chemicals called hormones to help your body function properly. The endocrine system completes these tasks through its network of glands that produce, store, and secrete hormones. In the previous subchapter, we discussed endocrine glands such as the thyroid, parathyroid, pancreas, ovaries, and testes. This subchapter will focus on how the hypothalamus and pituitary gland work together to produce and release hormones to elicit an effect on target organs/tissues to maintain homeostasis. This subchapter will also detail the control mechanisms of each hormone and the clinical effects of prolonged unbalance. It is important to note that this subchapter contains content that is not always covered in HK*2810. Although it is recommended that you use this subchapter as supplementary material, most of the information you will find will not be tested during this course.
Learning Outcomes
In this section you will learn…
- The functional relationship between the pituitary gland and the hypothalamus.
- How the anterior and posterior pituitary hormones are synthesized and released.
- The physiological impact of hormones on target organs/tissues.
- The control mechanisms (negative or positive feedback) by which the hypothalamus and pituitary are regulated.
The Hypothalamus
The hypothalamus is a small structure at the base of the brain near the pituitary gland that plays a crucial role in maintaining homeostasis. Typically the structures of the brain are protected from harmful solutes due to the blood-brain barrier. Even though the hypothalamus is located in the brain, it has a specialized blood-brain barrier that allows the dynamic passage of hormones and nutrients from the blood into the hypothalamus. This specialization of the blood-brain barrier allows the hypothalamus to sense hormonal imbalances throughout the body. Additionally, the hypothalamus being located at the base of the brain, can also receive information from different areas within the brain. Within the brain, the hypothalamus receives input from the thalamus, limbic system (emotion), optical system (vision), and reticular substances (sleep/wake cycle). </span>Once the hypothalamus receives and integrates information regarding an imbalance from the brain and the periphery it can communicate to the appropriate gland to restore homeostasis.
After the hypothalamus senses a change one of the main ways it is able to elicit a systemic response to restore homeostasis is through the hypothalamus-pituitary axis (HPA). The HPA describes the direct influences and feedbacks between the hypothalamus and pituitary gland to restore homeostasis. For now, in a simplistic sense, the hypothalamus communicates via nerve impulses and hormones with the pituitary gland. The hypothalamus and pituitary gland are located in close proximity to each other just above the brainstem, which allows for easy communication. To help you appreciate the proximity of these structures within the brain take a moment to analyze the learning object below.
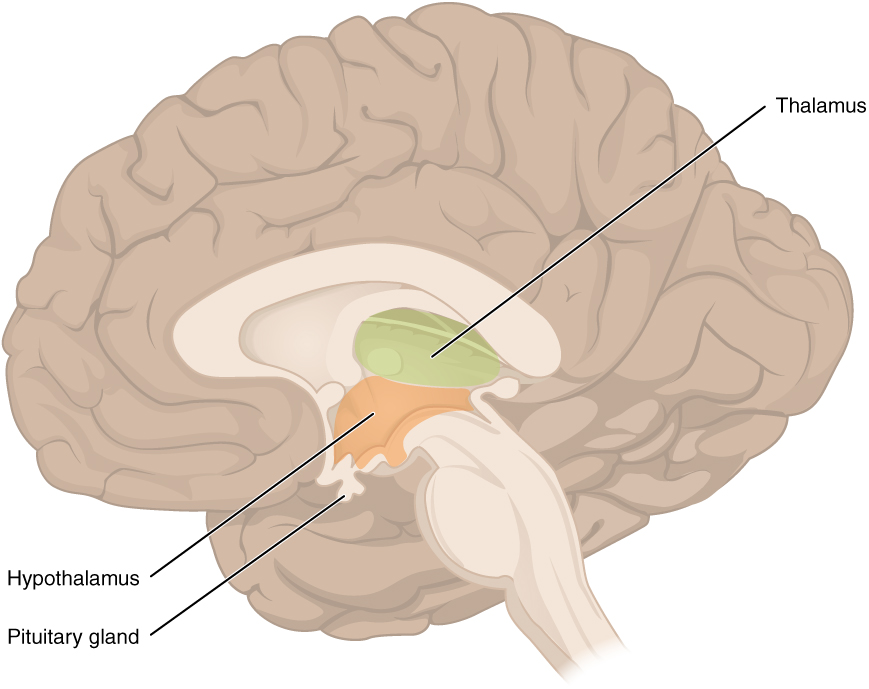
The Pituitary Gland
As mentioned previously, the hypothalamus is able to sense changes within the body and can communicate to the appropriate gland to restore homeostasis. Focusing on the HPA the gland that the hypothalamus communicates with via nerve impulses and hormones is the pituitary gland. The pituitary gland is a pea-sized gland located at the base of the hypothalamus. The hypothalamus and pituitary gland are connected by a structure called the infundibulum or sometimes referred to as the pituitary stalk. Following the direction of the hypothalamus, the pituitary gland is responsible for producing and releasing hormones to restore homeostasis. The pituitary gland releases hormones that interact with target tissues and organs such as the bone, breasts, ovaries, uterus, testis, adrenal cortex, thyroid gland, and kidneys. The pituitary gland consists of two lobes: the anterior lobe and the posterior lobe. Each lobe is under hypothalamic control to release hormones into systemic circulation; however, each lobe is stimulated to do this differently. The hypothalamus controls the anterior lobe by releasing hormones through the connecting blood vessels and it controls the posterior lobe through nerve impulses. Note the location of the hypothalamus and pituitary, and consider the anatomical and functional relationship. Once again to help you appreciate the proximity of these structures within the brain take a moment to analyze the learning object below
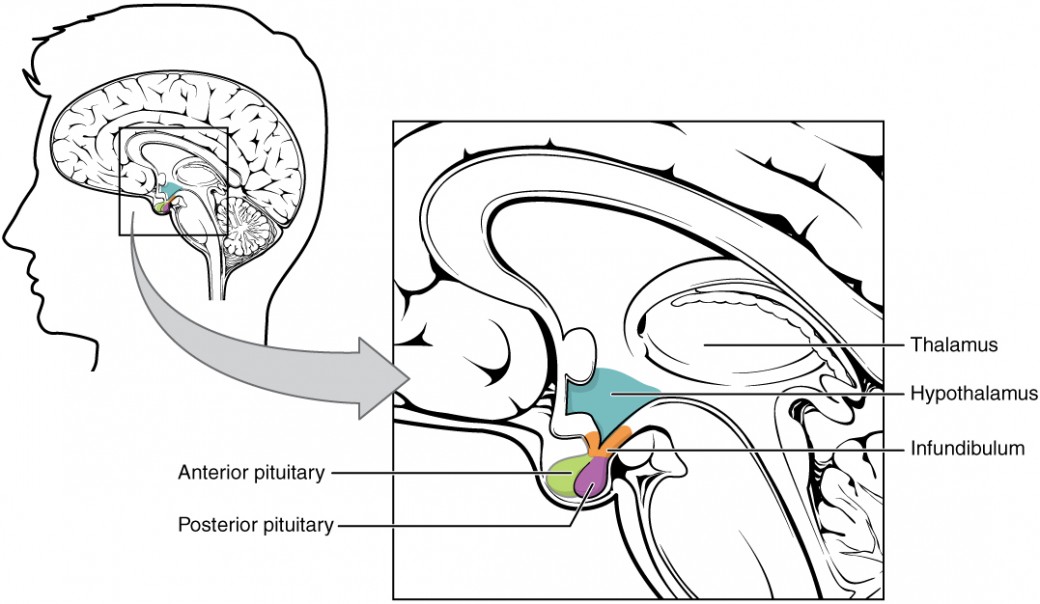
The Posterior Pituitary
The posterior pituitary which is also called the neurohypophysis, is essentially an extension of the hypothalamus. It’s important to note that the posterior pituitary cannot synthesize its own hormones rather it secretes hormones produced by the neurons in the hypothalamus. Recall that the hypothalamus communicates with the posterior pituitary lobe through nerve impulses. More specifically, specialized neurons from the hypothalamus have axons that project directly onto the posterior pituitary. When the hypothalamus senses changes within the body these neurons release hormones directly into the hypophyseal portal system. The hypophyseal portal system is the circulatory system within the infundibulum that connects the hypothalamus and the pituitary gland. The released hormones eventually reach the microvasculature of the posterior pituitary where they can be stored, or secreted into the systemic circulation. The two hormones this subchapter will discuss that are secreted by the posterior pituitary are oxytocin and antidiuretic hormone (ADH). The learning object below takes a closer look into the proximity and the connectivity between the hypothalamus and the posterior pituitary. Notice the pathway of neurons from the hypothalamus through the hypothalamohypophyseal tract to release oxytocin (blue) and ADH (red) into the hypophyseal portal system. Once in the hypophyseal portal system, oxytocin and ADH can be stored or released by the posterior pituitary.
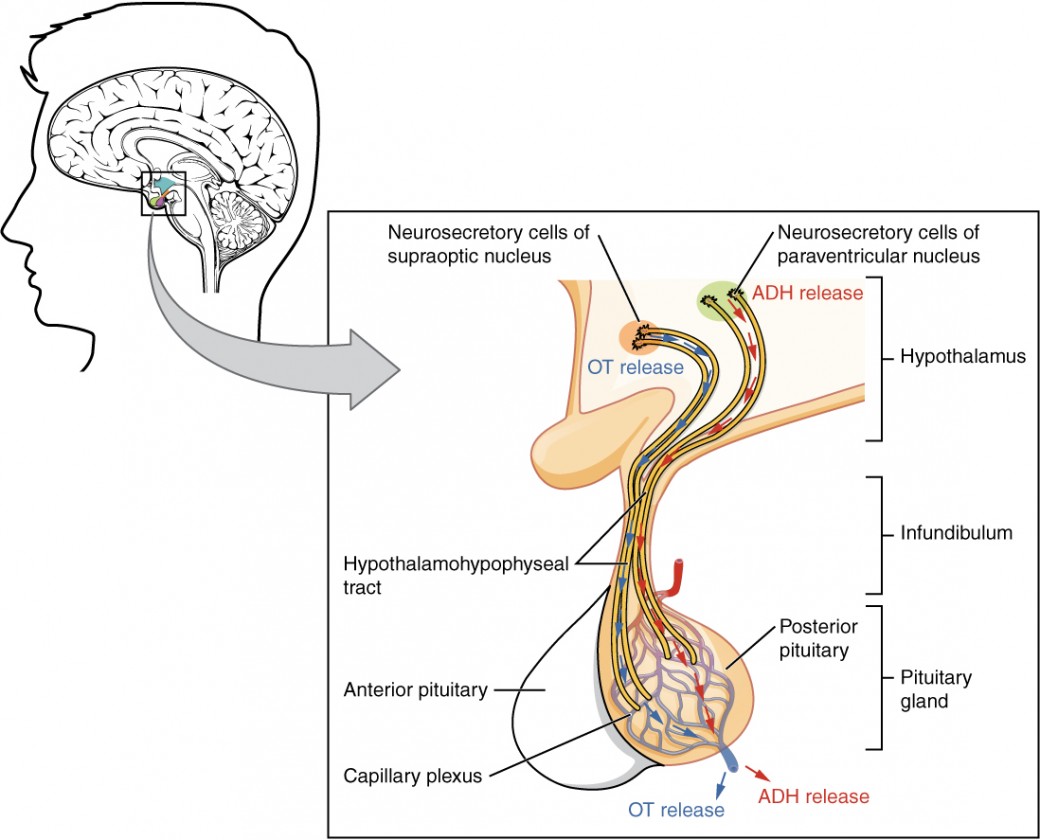
Before reading any further take a moment to analyze the table below. The table below is an excellent study tool that summarizes the target tissue and physiological roles of the hormones produced by the hypothalamus and secreted by the posterior pituitary.
Posterior Pituitary Hormone | Oxytocin | Antidiuretic hormone |
---|---|---|
Target Tissue | Uterus and mammary glands | Kidneys |
Physiological Roles | Stimulates uterine contractions during childbirth | Raise blood pressure or decrease blood osmolarity |
Oxytocin
Oxytocin is a peptide hormone released systemically by the posterior pituitary pregnancy and childbirth. Oxytocin is maintained at low levels during pregnancy, but towards the end of pregnancy, the uterus becomes more sensitive to oxytocin by upregulating oxytocin receptors. The upregulation of oxytocin is critical in childbirth because it initiates a positive-feedback mechanism that ends in the delivery of the baby.[1] During childbirth, uterine contractions will push the baby towards the cervix stretching it in the process. The cervix has nerve cells that act as stretch receptors that transmit this information to the brain. Cervical stretching stimulates the hypothalamus to produce oxytocin, which in turn, acts on the oxytocin receptors on the uterus to increase the strength of the contractions.[2] As labor progresses and the cervix will continue to stretch, more oxytocin will be released, and the population of oxytocin receptors will increase creating positive feedback. Once the baby is fully delivered, cervical stretching will cease and stops being perceived by the hypothalamus, terminating positive feedback.[3]
When oxytocin binds to its receptor, the following reaction ensues:
- Oxytocin binds to the oxytocin receptor on uterine smooth muscle.
- This causes G-protein dissociation.
- Phospholipase C (PLC) produces IP3.
- IP3 causes calcium release from intra-cellular stores.
- Calcium release stimulates uterine contraction.
While oxytocin levels decrease after birth, they still play an essential role in child and maternal health. In response to a suckling newborn, sensory receptors in the nipple transmit signals to the hypothalamus to secrete oxytocin. Oxytocin acts at the mammary gland allowing breast milk to flow through the ducts, for ejection out of the nipple.[4]
Note this is an important concept and you should be able to describe this feedback mechanism in detail. This is a hallmark example of positive feedback and is depicted in the diagram below.
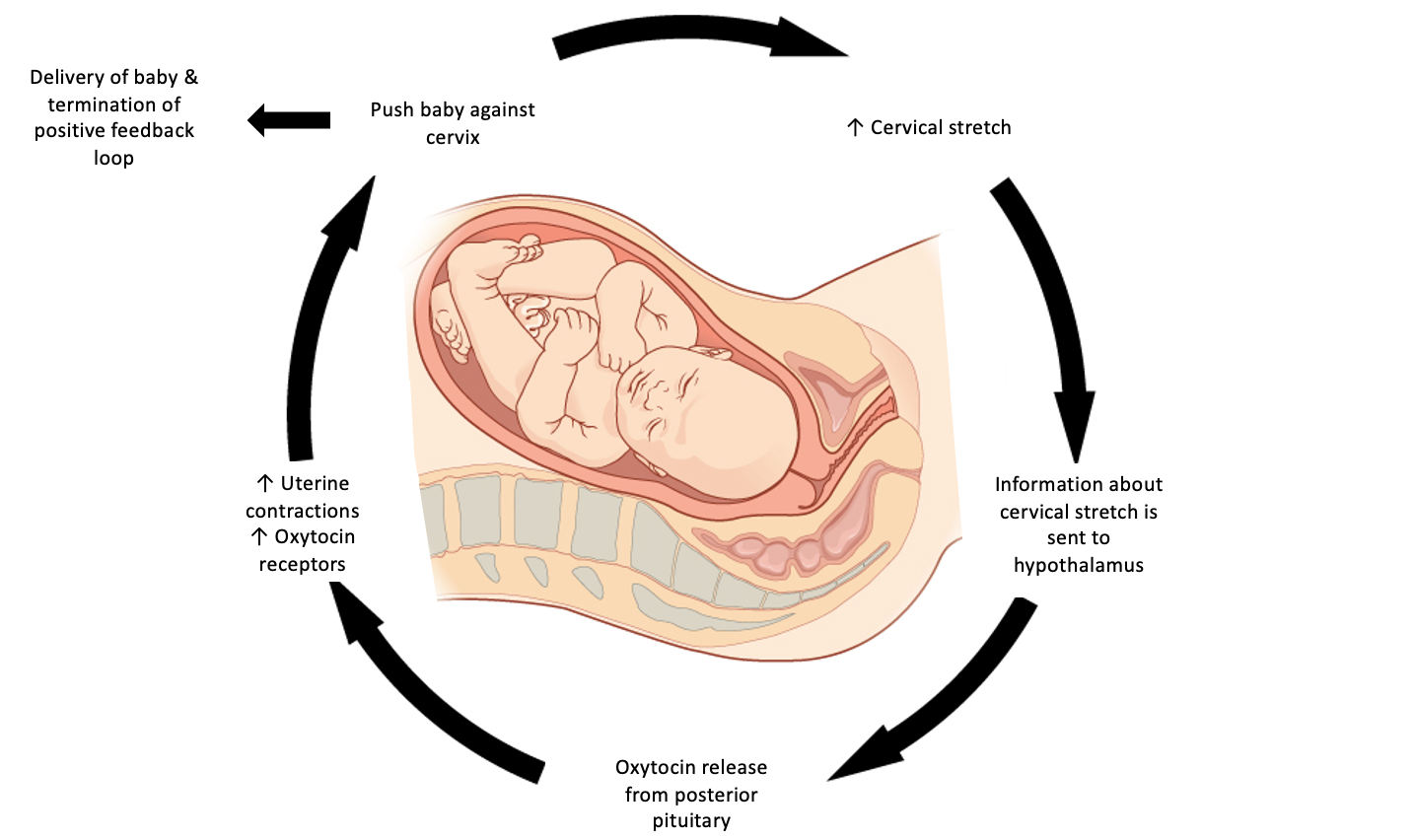
Antidiuretic Hormone (ADH)
Antidiuretic hormone is a peptide hormone released systemically by the posterior pituitary. There are two stimuli for ADH release:
1. An increase in blood osmolarity
2. A decrease in blood pressure
Specialized receptors on the hypothalamus called osmoreceptors, can sense a change in blood osmolarity and stimulate the release ADH from the posterior pituitary. When someone has low blood pressure, stretch receptors in the vasculature decrease their action potential, frequency and pattern in response to decreased stimulus/stretch. This information is transmitted to the brain stem and causes ADH release from the posterior pituitary.
ADH has two targets: the kidney and vascular smooth muscle. At the kidney, ADH stimulates water reabsorption which dilutes the high concentration of solutes to restore homeostatic osmolarity. At the vascular smooth muscle, ADH stimulates vasoconstriction to increase blood pressure.
This system works via negative feedback, meaning that once ADH restores homeostatic levels of osmolarity and blood pressure, it will be sensed by osmoreceptors in the hypothalamus, to stop secreting ADH. Stretch receptors in blood vessels will increase their action potential, frequency and pattern, inhibiting release of ADH, as well.
Ever wonder what causes a hangover? Consumption of drugs or alcohol can interfere with the secretion of ADH release and cause increased urine output. Large urine outputs eventually lead to dehydration and symptoms that may collectively be referred to as a “hangover”.[5]
Are you interested in learning more about conditions caused by low ADH? Diabetes insipidus is a condition caused by the body’s inability to either properly produce or respond to ADH. Low ADH in the blood means that the kidneys are unable to retain water. Consequently, this condition is associated with excessive urine output, extreme thirst and dehydration. There are two main types of diabetes insipidus: central diabetes insipidus and nephrogenic diabetes insipdus. Central diabetes insipidus refers to problems relating to the pituitary gland that cause this disorder. Damage to the pituitary gland, such as tumours, brain injuries or surgeries, can interfere with its ability to produce ADH. Nephrogenic diabetes insipidus refers to the structural changes that happen in the kidney to cause this disorder. While this form of diabetes insipidus can be inherited, it is also seen in people with other kidney diseases and as a side-effect of certain drugs. The mechanism of this nephrogenic diabetes has not been scientifically confirmed, however the structural changes in the tubules of the kidney associated with some of these conditions leave the kidney unable to respond the ADH. [6]
The Anterior Pituitary
The anterior pituitary which is also called the adenohypophysis, is a true endocrine gland and receives direction from the hypothalamus to secrete a hormone into systemic circulation. This ‘direction’ comes in the form of hypothalamic releasing hormones. Hypothalamic releasing hormones are released from the hypothalamus in response to a stimulus, and are secreted into the hypophyseal portal system. Once the releasing hormone reaches the microvasculature of the anterior pituitary, it is sensed by endocrine cells. The anterior pituitary is stimulated to produce and secrete a secondary hormone, and releases it into systemic circulation to act on target tissues. The anterior pituitary produces seven hormones of which their functions are summarized in the table below:
Releasing Hormone from Hypothalamus | Prolactin-releasing hormone (PRH) | Growth hormone releasing hormone (GHRH) | Gonadotropin releasing hormone (GnRH) | Gonadotropin releasing hormone (GnRH) | Thyrotropin-releasing hormone (TRH) | Corticotropin releasing hormone (CRH) |
---|---|---|---|---|---|---|
Anterior Pituitary Hormone | Prolactin | Growth Hormone | Luteinizing Hormone | Follicle Stimulating Hormone | Thyroid Stimulating Hormone | Adrenocorticotropic Hormone |
Target Tissue | Ovaries and mammary glands | All tissues | Gonads | Gonads | Thyroid Gland | Adrenal Gland |
Physiological role | Milk production, estrogen and progesterone production | Promotes growth and metabolism | Sex hormone production | Reproductive system maintenance | Thyroid hormone production | Production of glucocorticoids, mineralocorticoids and androgens |
Growth Hormone
The hypothalamus releases growth hormone (GH), which is also called somatropin, from the anterior pituitary. In childhood, GH stimulates post-natal growth and development. In adulthood, it maintains lean body and bone mass. Growth hormone is named for its overall function: enabling growth of bone and tissue. Consequently, higher levels of growth hormone are seen when humans are sleeping or at rest, a time where energy demand is low and energy reserves can be used for growing.
GH is an anabolic peptide hormone that works through direct and indirect mechanisms to promote protein and tissue synthesis. Where direct mechanisms describe the effect of GH on tissues, indirect mechanisms describe the effect of insulin-like growth factors (IGFs) on tissues. Most IGFs are produced and then used locally in an autocrine or paracrine manner. The exception to this rule, is the production of IGFs in the liver. The liver secretes IGFs into systemic circulation to act in an endocrine manner.
Growth hormone releasing hormone (GHRH) is released from the hypothalamus in response to a stimuli, which acts on the anterior pituitary to release GH. Somatostatin, also called growth hormone-inhibiting hormone (GHIH), is released from the hypothalamus and inhibits GH release from the anterior pituitary.
GH release from the anterior pituitary will directly affect adipose tissue and stimulate lipolysis, increasing the liberation of free fatty acids (FFA) causing decreased adiposity. At the skeletal muscle, GH will decrease glucose uptake and increase amino acid uptake. This increases protein synthesis and thereby lean body mass. GH also acts directly acts on the liver to increase gluconeogenesis and IGF production. IGFs increase protein synthesis and acts to increase cell size and cell number in many organs (heart, lungs, bone, connective tissue). This translates to an increase in organ size and function over time.
Target Tissue | Effect of Growth Hormone |
---|---|
Adipose | -decrease glucose uptake
-increase lipolysis -increase free fatty acids -decrease adiposity |
Liver | -increase gluconeogenesis
-increase IGF production |
Skeletal Muscle | -decrease glucose uptake
-increase amino acid uptake -increase protein synthesis |
GH is heavily controlled by negative feedback mechanisms. GHRH exhibits negative feedback on the hypothalamus. GH exhibits negative feedback on the anterior pituitary. IGFs exhibits negative feedback on the hypothalamus and anterior pituitary.
The diagram below shows a visual representation of how growth hormone and IGF release is regulated and the effect they have on target tissues.
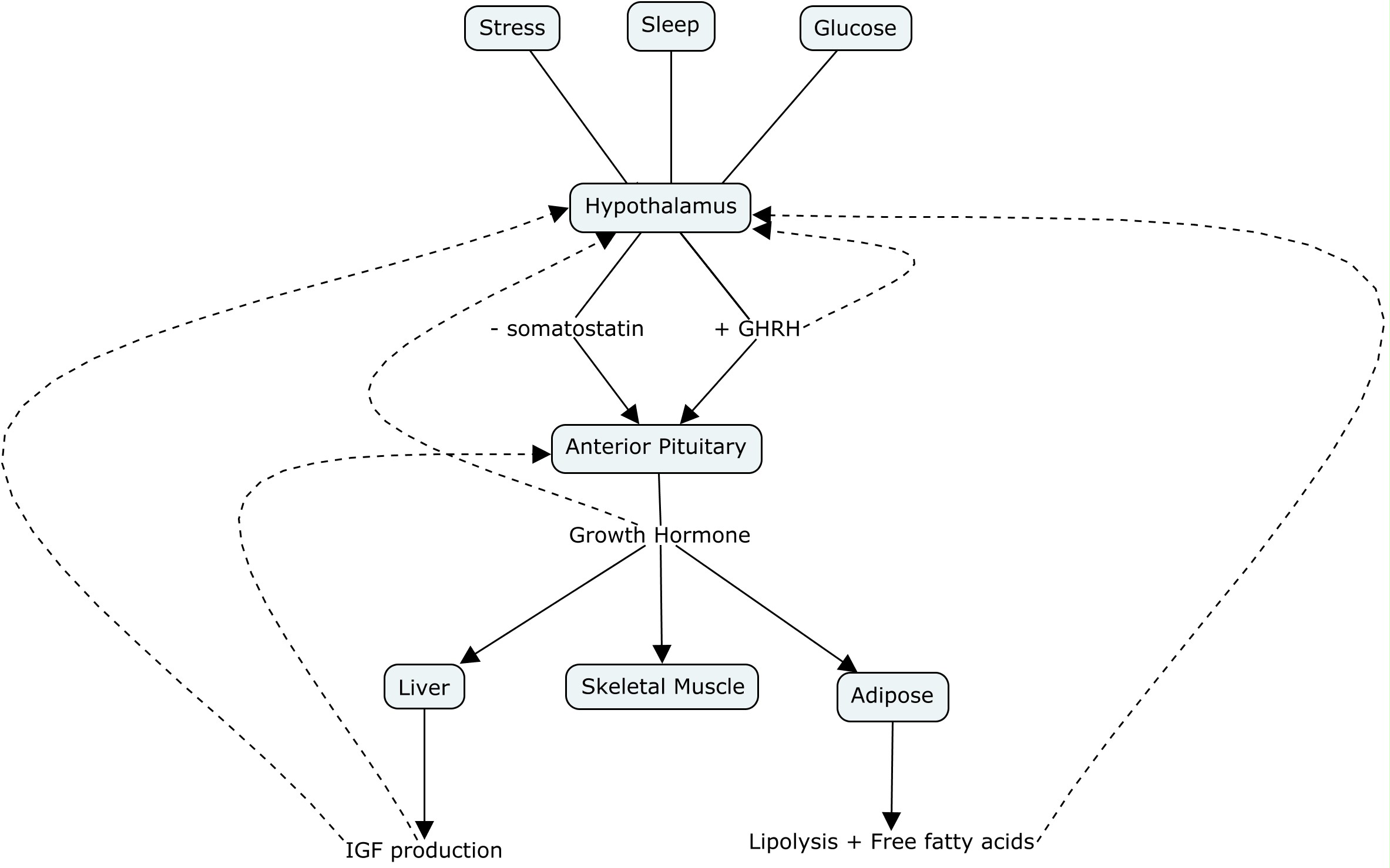
Did you know there are several disorders caused by disrupted levels of growth hormone? Gigantism is a rare disorder diagnosed in children caused by excessive growth hormone secretion. It is usually caused by a benign tumour on the anterior pituitary gland (pituitary adenomas) that increases GH secretion. Because this happens in childhood before growth plates have fused, excessive GH causes extreme height, organ and muscle size. Gigantism is usually treated by surgically removing the tumour, however, if there is risk of physical damage to arteries or nerves, medication may be used to shrink the tumour.[7]
Acromegaly is a rare disorder that occurs in adults and is caused by excessive growth hormone secretion. Like gigantism, acromegaly is also caused by a benign tumour on the anterior pituitary. Because growth plates are already fused in adults, the effects of excessive growth hormone production are different than in children. Rather, adults with acromegaly is associated with robust features like enlargement of the hands and feet, and facial features. Like gigantism, acromegaly is usually treated with surgery, or with medication when surgery is contraindicated.[8]
Pituitary dwarfism can be a congenital or acquired condition caused by GH deficiency. In children, pituitary dwarfism causes below average height, but maintains average proportions and is often idiopathic. In adults, GH deficiency causes reduced muscle and bone mass and decreased energy, among others. In children, GH deficiency is treated by daily injections of GH. In adults, it is treated with daily injections of growth hormones, at a much lower dose than what children would receive.[9]
Thyroid Stimulating Hormone
The hypothalamus releases thyrotropin-releasing hormone (TRH) which acts on the anterior pituitary to produce thyroid-stimulating hormone (TSH). TSH acts on the thyroid gland to stimulate triiodothyronine (T3) and thyroxine (T4) secretion into the blood. 75% of T4 is converted to its active form, T3, at the level of the cell and is thus considered a prohormone. T4 and T3 are lipid soluble hormones and circulate bound to proteins in the blood.
The structures of T4 and T3 are very similar and differ by only one iodine atom, where T3 is the active form. These structures are shown in the following image. You are not expected to know their structures, just realize that they are very similar.
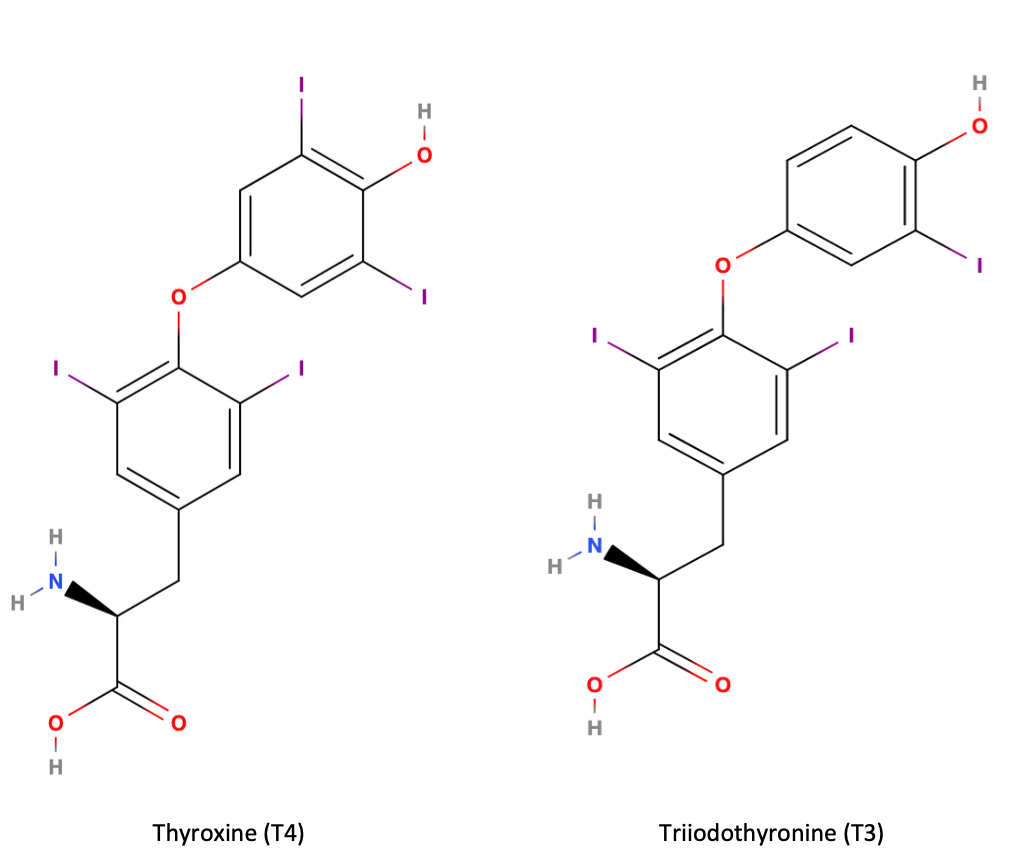
Unbound thyroid hormone is biologically active in the blood and has a very short half life (minutes) whereas bound thyroid hormone is inactive and has a longer life (days). Consequently, protein-bound thyroid hormone acts as a reservoir for T3 and allows for strict control over T3 levels; the reservoir is always available but only accessed when required. When homeostatic concentrations of T3/T4 are achieved, this is sensed by the anterior pituitary and hypothalamus, which will stop secreting TSH and TRH, respectively.
T3/T4 enter the cell bound to a carrier protein, where T4 will be converted to T3. Once in the cell, T3 will bind to its nuclear receptor to increase mRNA expression of Na+/ATPase pumps and to increase the number of mitochondria. Why is this significant? Close to 50% of our daily caloric intake is dedicated to powering Na+/ATPase pumps. Increasing the number of pumps, increases metabolism and thereby increases caloric demand. Further, increasing the number of mitochondria and associated respiratory enzymes, will increase the rate of ATP synthesis and thereby oxygen consumption. This is beyond the scope of this course, but an increase in oxygen consumption increases metabolic rate. Overall, the physiological role of T3/T4 is to induced changes at the nuclear level to increase metabolism.
The diagram below shows how T3/T4 enters the cell through the T3 transporter and acts at the nucleus to alter gene expression. Not shown in this diagram is the conversion of T4 to T3, when T4 enters the cell.
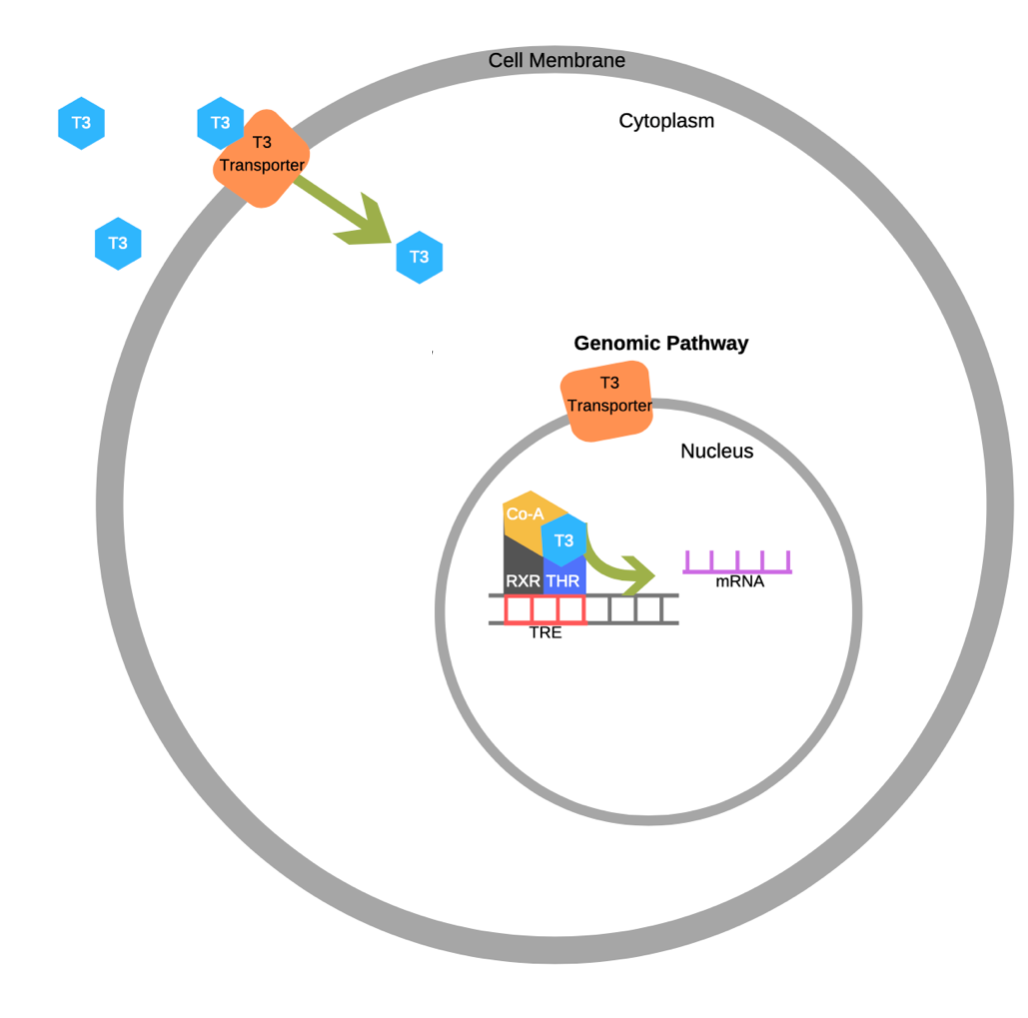
T3/T4 control cellular metabolism, where high concentrations in the blood will increase metabolism and low concentrations will decrease metabolism. Consequently, things that alter metabolism like food intake and body temperature will also alter T3 and T4 hormone secretion. For example, exposure to cold will stimulate TSH release and increase metabolism, which increases body temperature; fasting will decrease metabolism and conserve energy reserves; growth hormone inhibits TSH release, and slows metabolism conserving energy reserves that can be allocated for growing.
Ever wonder what regulates thyroid hormone secretion in the thyroid? In previous sections you learned that glucose levels causes fluctuations in insulin and glucagon secretion from the pancreas. However, in the thyroid, the regulated variable that regulates thyroid hormone secretion is unknown. We know certain things influence thyroid hormone production (body temperature, circadian rhythms and stress), but we have no idea why!
The following diagram demonstrates how T3/T4 release is regulated and the effect they have on target tissues.
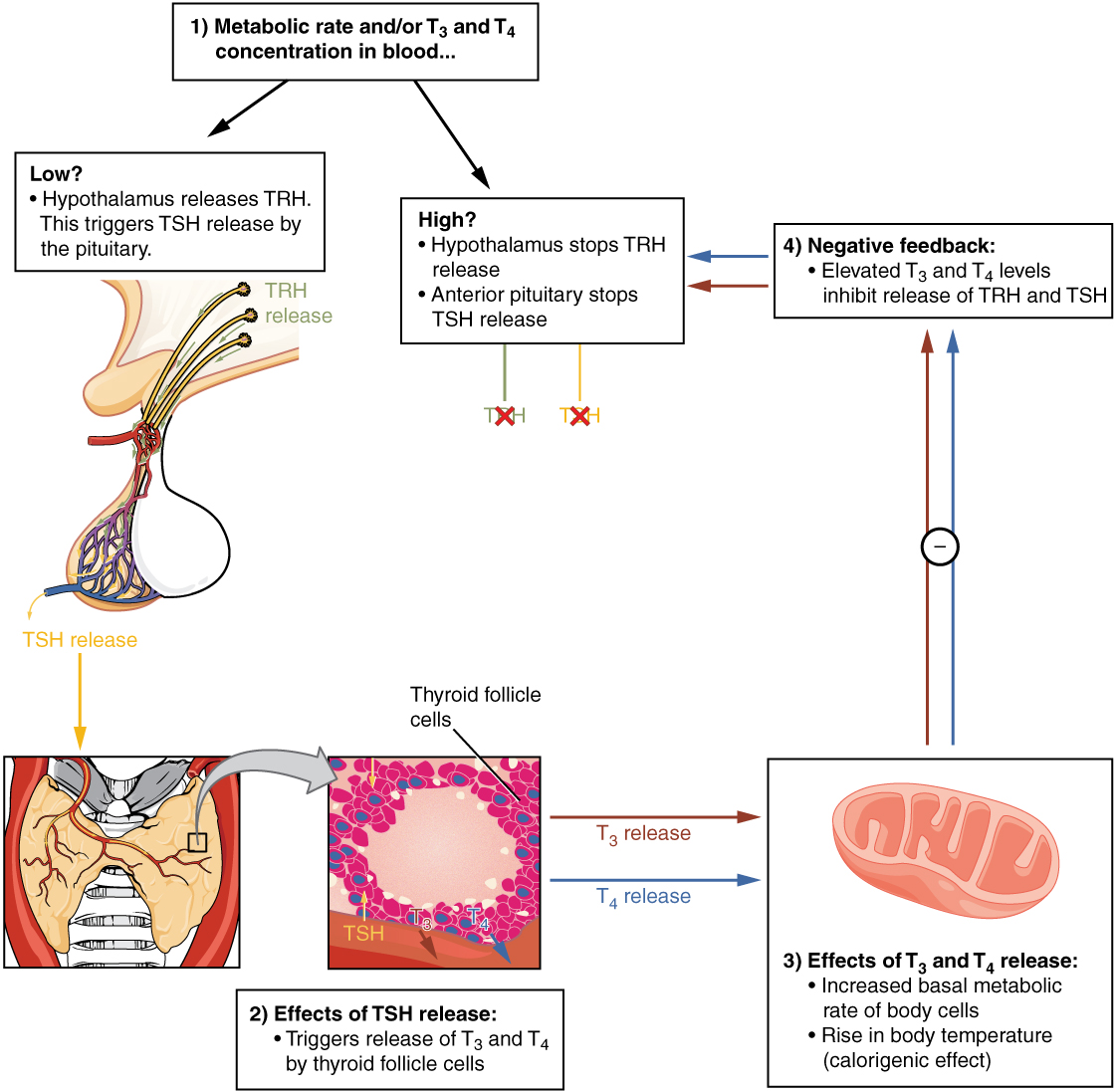
Are you interested in learning more about thyroid disorders? Hyperthyroidism is caused by overproduction of thyroxine and accelerates metabolism. Symptoms of hyperthyroidism include, intolerance to heat, weight loss, fatigue and a goitre. Hyperthyroidism has many causes such as infection causing inflammation of the thyroid (thyroiditis), autoimmune disease (Grave’s disease), and thyroid tumours. Treatment varies depending on the cause of disease; however most can be treated without surgery. Prescribed medication commonly targets the enzyme that produces thyroid hormone to inhibit T3/T4 production. Other drugs are sometimes prescribed to relieve symptoms caused by hyperthyroidism, but do not treat the cause of disease.[10]
Hypothyroidism is caused by insufficient thyroid hormone production, and slows metabolism. Symptoms of hypothyroidism are generally opposite of hyperthyroidism such as intolerance to cold and weight gain; however, both diseases are commonly associated with fatigue and goitre. Hypothyroidism is usually caused by an autoimmune disease (Hashimoto’s thyroiditis) or by treatment of hyperthyroidism. Overzealous treatment of hyperthyroidism with medication that inhibits T3/T4 production can cause hypothyroidism. Treatment of hypothyroidism includes daily supplementation of synthetic thyroid hormone or lowering the dose of hyperthyroid medication if its caused by medication.[11]
Adrenocorticotropic Hormone
The hypothalamus releases cortiocotropic-releasing hormone (CRH) in response to stress. CRH reaches the anterior pituitary which triggers adrenocorticotropic hormone (ACTH) release by the anterior pituitary. ACTH then travels to the adrenal glands where it triggers cortisol release. Cortisol has a variety of physiological effects mainly regulating metabolism, immunosuppressive and anti-inflammatory effects. Chronic stress can lead to prolonged elevated levels of cortisol which can have harmful physiological effects.
High cortisol levels are sensed by the hypothalamus and anterior pituitary to stop secreting CRH and ACTH, respectively. However, problems arise in states of chronic stress. Although, negative feedback mechanisms are in place to terminate cortisol production (i.e. negative feedback), stress will stimulate the cycle to continue, leading to chronically elevated cortisol levels.[12]
Follicle-Stimulating Hormone and Luteinizing Hormone
The hypothalamus releases gonadotropin releasing hormone (GnRH) which acts on the anterior pituitary to secrete FSH and LH. These hormones have different target organs in males and females and are regulated in similar but different ways. This was explained in more detail in earlier sections.
In females:
Follicular Phase:
In females, FSH and LH will act on the ovary to enhance follicle recruitment and growth. As follicles continue to grow, they will produce low levels of estradiol to support the endometrium. Estradiol will feedback on the hypothalamus to inhibit GnRH secretion, which in turn suppresses FSH and LH release. Low levels of FSH and LH causes endometrial arterioles to constrict and induce menstruation.
Ovulation Phase:
FSH and LH stimulates the maturation of one follicle. As the follicle grows, it will produce high levels of estradiol. High levels of estradiol positively feedback on the hypothalamus which induce GnRH secretion, stimulating the anterior pituitary to secrete FSH and LH. Surges of LH induces the follicle to rupture (ovulation) one day later.
Luteal Phase:
LH acts on the ruptured follicle to form a corpus luteum. The corpus luteum will produce progesterone and estradiol which inhibits GnRH secretion at the hypothalamus and FSH and LH secretion at the anterior pituitary. Lack of FSH and LH causes the corpus luteum to degrade. Fertilization of the egg prevents degradation of the corpus luteum, and continues to secrete progesterone and estradiol to maintain pregnancy.
The LO below depicts the different hormones that are secreted during the different phases of the menstrual cycle. Hormones play an important role in preparing the body for pregnancy.
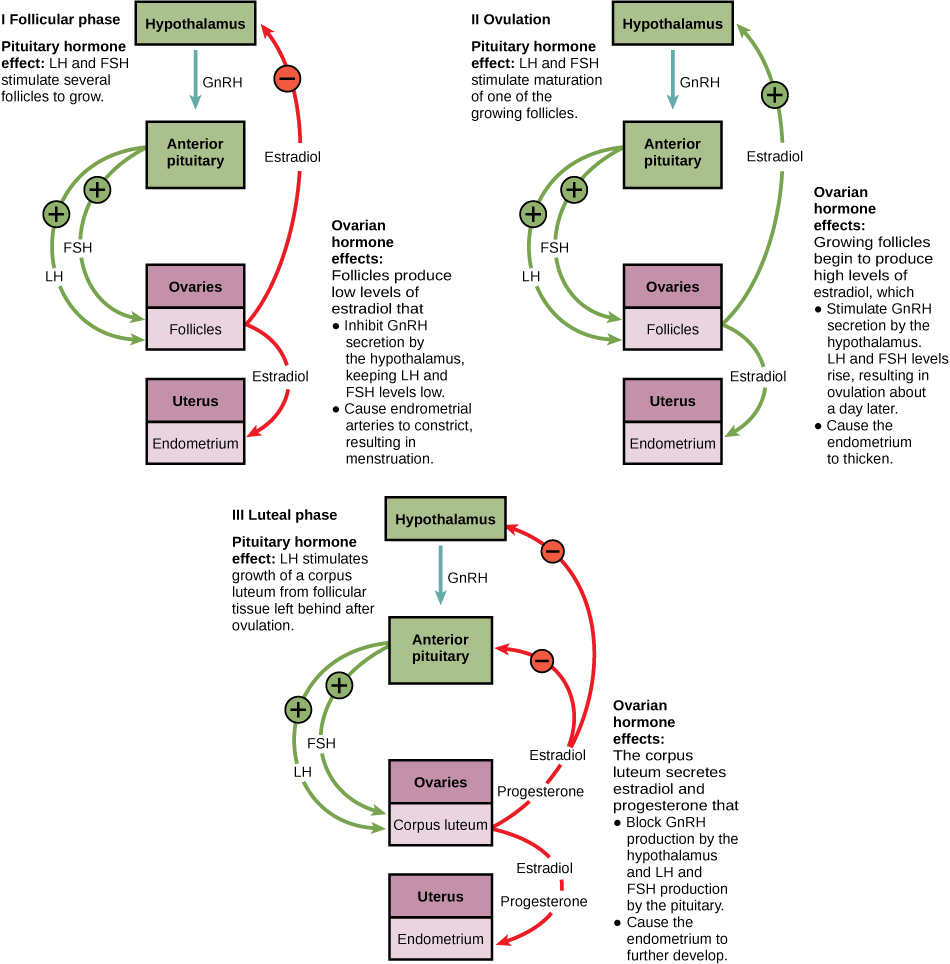
In males:
In males, FSH acts on Sertoli cells to stimulate spermatogenesis and LH acts on Leydig cells to cause testosterone secretion. High testosterone causes negative feedback at the hypothalamus and anterior pituitary to stop GnRH secretion. Inhibin secretion by Sertoli cells also exhibits negative feedback on the anterior pituitary. Tight regulation over testosterone production is critical to maintaining an optimal environment for sperm maturation. A visual representation of the hormones involved in spermatogenesis are depicted in the diagram below.
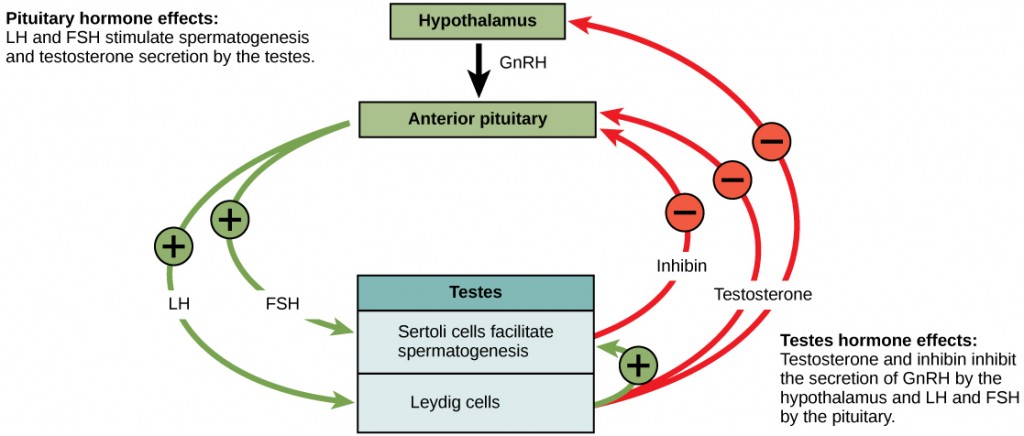
Prolactin
Prolactin is a hormone secreted by the anterior pituitary and promotes lactation in women. During pregnancy prolactin contributes to the development of mammary glands, and the production of breast milk after childbirth. After childbirth, prolactin levels sharply decline, but increase during breastfeeding. The effects of prolactin occur only under the permissive effects of other hormones sex hormones and oxytocin. [13]
Whereas other anterior pituitary hormones are under hypothalamic control by releasing hormones, the presence of a prolactin-releasing hormone has only been hypothesized. While there are hormones that stimulate the release of prolactin like oxytocin, estrogen and thyrotropin releasing hormone, these hormones have other primary functions. Rather, prolactin release from the anterior pituitary is controlled by inhibitory regulators. For example, dopamine released from the hypothalamus prevents the release of prolactin from the anterior pituitary.[14]
Did you know hyperprolactinemia is a disorder that causes high levels of prolactin in the blood? This can occur in both men and women and is caused by pituitary tumours, hyperthyroidism and some medications. High levels of prolactin in both men and women who are not pregnant can cause breast milk production. High levels of prolactin also decrease sperm production in men and can lead to difficulties conceiving for women. Treatment of hyperprolactinemia depends on the cause of the disease. Hyperprolactinemia is usually treated with medication to either shrink a pituitary tumour, or to control hyperthyroidism. Sometimes treatment is not required if the women is still producing estrogen.[15]
Key Takeaways
Consider the following concepts to help guide your studies:
- How the hypothalamus is important for maintaining homeostasis in the body by communicating to the pituitary.
- Compare and contrast the anterior and posterior pituitary.
- Know which hormones are secreted by which lobe and what they target.
The questions below can be used to assess your knowledge within this chapter. There are multiple-choice questions that you should attempt without referring to your notes. The questions will provide you with responses to your answers to guide your studying but should not be used as your only resource.
Subchapter Quiz
Media Attributions
- Anatomy of the Hypothalamus-Pituitary Axis is licensed under a CC0 (Creative Commons Zero) license
- Anatomy of structures involved in the Hypothalamus Pituitary Axis is licensed under a CC0 (Creative Commons Zero) license
- Posterior Pituitary Hormone Release is licensed under a CC0 (Creative Commons Zero) license
- Oxytocin feedback adapted by Jennifer St. Amant is licensed under a CC0 (Creative Commons Zero) license
- Growth Hormone Action on Tissues is licensed under a CC0 (Creative Commons Zero) license
- Thyroid Hormones adapted by Jennifer St. Amant
- Thyroid hormone action at the nucleus © Arizzo0226 adapted by Jennifer St. Amant is licensed under a CC BY-SA (Attribution ShareAlike) license
- Thyroid hormone regulation is licensed under a CC0 (Creative Commons Zero) license
- The Negative Feedback Loop of the Ovarian Cycle © Lumen Learning is licensed under a CC0 (Creative Commons Zero) license
- Hormonal Regulation of Sperm Production © Molnar, C. and Gair, J. is licensed under a CC0 (Creative Commons Zero) license
- Betts, J.G., Young, K.A., Wise, J.A., Johnson, E., Poe, b., Kruse, D.H., Korol, O., Johnson, M.W., DeSaix, P. (2013). The Pituitary Gland and Hypothalamus. Openstax. ↵
- Betts, J.G., Young, K.A., Wise, J.A., Johnson, E., Poe, b., Kruse, D.H., Korol, O., Johnson, M.W., DeSaix, P. (2013). The Pituitary Gland and Hypothalamus. Openstax. ↵
- Betts, J.G., Young, K.A., Wise, J.A., Johnson, E., Poe, b., Kruse, D.H., Korol, O., Johnson, M.W., DeSaix, P. (2013). The Pituitary Gland and Hypothalamus. Openstax. ↵
- Betts, J.G., Young, K.A., Wise, J.A., Johnson, E., Poe, b., Kruse, D.H., Korol, O., Johnson, M.W., DeSaix, P. (2013). The Pituitary Gland and Hypothalamus. Openstax. ↵
- Betts, J.G., Young, K.A., Wise, J.A., Johnson, E., Poe, b., Kruse, D.H., Korol, O., Johnson, M.W., DeSaix, P. (2013). The Pituitary Gland and Hypothalamus. Openstax. ↵
- Mayo Clinic Staff. (n.d.). Diabetes insipidus. Mayo Clinic. ↵
- McGurgan, H. (2018). Gigantism. healthline. ↵
- Mayo Clinic Staff. (n.d.). Acromegaly. Mayo Clinic. ↵
- Mayo Clinic Staff. (n.d.). Dwarfism. Mayo Clinic. ↵
- Nazario, B. (2021).Hyperthyroidism.WebMD. ↵
- Mayo Clinic Staff. (n.d.). Hypothyroidism. Mayo Clinic. ↵
- Adrenal Glands ↵
- Betts, J.G., Young, K.A., Wise, J.A., Johnson, E., Poe, b., Kruse, D.H., Korol, O., Johnson, M.W., DeSaix, P. (2013). Lactation. Openstax. ↵
- Betts, J.G., Young, K.A., Wise, J.A., Johnson, E., Poe, b., Kruse, D.H., Korol, O., Johnson, M.W., DeSaix, P. (2013). Lactation. Openstax. ↵
- Reproductivefacts.org. (2014). Hyperprolactinemia. ASRM. ↵
Small portion of the brain that regulates metabolic processes, serving as a link between endocrine and nervous systems via the pituitary gland.
A barrier between circulating blood and the CNS that is highly selective, allowing nutrients and small products to reach the brain while preventing damage and infection by blocking diffusion of larger products and pathogens.
An endocrine gland consisting of anterior and posterior portions that secrete hormones in response to hypothalamic stimuli.
Also known as the pituitary stalk, the infundibulum connects the hypothalamus to the pituitary gland.
Pituitary gland lobe that does not produce its own hormones. Hormones synthesized in the hypothalamus reach the posterior pituitary where they are secreted in systemic circulation.
The connection of blood vessels between the hypothalamus and pituitary gland.
Solutes in the blood.
Part of the endocrine gland and is known to produce its own hormones for secretion into systemic circulation.
Biologically active form of thyroid hormone.
Prohormone is converted to the biologically active form of thyroid hormone (T3) at target tissues.
The precursor to a hormone. A prohormone does not have a direct biological effect but may act as a reservoir for one that does and can be converted to a biologically active hormone.
Thyroid gland enlargement that causes neck swelling.