Pulmonary Mechanics and Respiration
For many of us, breathing is something we take for granted as we rarely notice we are even doing it. Breathing is typically regulated automatically by the body but can be controlled if we decide to override it in order to hold our breath or talk. We will now discover three mechanisms the human body uses for breathing. These include pressure relationships, inspiration, and expiration. Finally, we will go over some real-life examples and practice questions to test your knowledge of the respiratory system’s mechanics.
Learning Outcomes
In this section you will learn…
- How to explain the mechanism of breathing in relation to pleural pressure, alveolar pressure, and atmospheric pressure.
- The various pressure relationships within the respiratory system and how these pressures contribute to gas exchange.
- How to explain changes in lung volume during breathing.
- How to describe the varying lung volumes and capacities.
- About surface tension and the effects of surfactant in relation to breathing.
- How to apply knowledge of pulmonary mechanics and respiration to real-life scenarios.
Pressure Relationships
The difference in atmospheric pressure and pressure in the lungs is what results in inhalation and exhalation. In order to understand the mechanisms of breathing, we must first understand pressure relationships. The relationship between pressure and volume can be explained by Boyle's Law, which states that, at a constant temperature, the volume of a fixed amount of gas is inversely proportional to its pressure, as seen in figure 1. If volume decreases within the system, then pressure increases, and if volume increases, then pressure decreases. The following formula expresses Boyle’s Law, where P1 represents the initial pressure, and V1 represents the initial volume. Likewise, the final pressure and volume are defined by P2 and V2, respectively.
[latex]P_1V_1=P_2V_2[/latex]
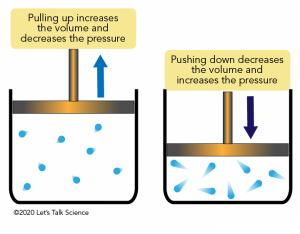
Applying Boyle’s Law to Respiration
Boyle’s law can be applied to any closed system. Imagine holding an inflated balloon; the pressure inside the balloon is the same as the atmosphere’s pressure. This will be similar to our lung volume before inspiration. Now, imagine you stretch that balloon and increase its volume. What will happen to the pressure? The pressure will decrease as a result of the balloon being extended to a larger volume. During inspiration, the lungs stretch, increasing volume, which results in a decrease in pressure. The higher-pressure atmospheric air will then move into the lungs until the pressures equilibrate. When exhaling, the lung volume decreases, and pressure increases. Thus, the air will leave the lungs and return to the now lower atmosphere pressure until the pressures equilibrate again. Overall, as the lungs expand and increase volume, inspiration occurs, and air flows in, as seen in figure 2. As the volume inside the lungs decreases during expiration, the air flows out according to its pressure.

Test Your Knowledge
Real-Life Scenario:
How would Boyle’s Law explain the change in ventilation, either inspiration or expiration, when you are hiking up a large mountain, meaning you are at a higher elevation. Hint: Think about how pressure changes at elevation and which pressures would be P1 and P2 within this scenario.
Pressure Changes
Four types of pressure are associated with breathing and are important to discuss before diving into pressure changes and how they allow for breathing. Atmospheric pressure is the air pressure in the atmosphere at sea level, 760 mmHg. All other lung pressures are expressed relative to this pressure for simplicity. Intra-alveolar or alveolar pressure is the air pressure inside the lung alveoli and varies during ventilation. Intrapleural or pleural pressure is the air pressure within the pleural cavity and varies during ventilation. Lastly, transpulmonary pressure is the difference between pleural pressure and alveolar pressure. Under physiological conditions, this pressure is always positive, as shown in figure 3.
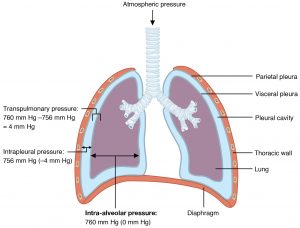
You’ll notice that the values in figure 3 do not correlate with the stated atmospheric pressure, which is 760 mmHg. Let’s dive into this concept now. Recall that functional residual capacity, otherwise known as normal breathing atmospheric pressure, is 760 mmHg and remains constant throughout the breathing process. Alveolar pressure is 760 mmHg and pleural pressure is 756 mmHg. If you remember from earlier, lung pressures are expressed relative to the atmospheric pressure. So, if we set the atmospheric pressure to 0 mmHg, then the alveolar pressure and pleural pressure can also be defined as 0 mmHg and −4 mmHg, respectively.
The pleural pressure and alveolar pressure vary with ventilation. However, under normal physiological conditions, the pleural pressure will always be negative and less than the alveolar pressure. The negative pleural pressure is due to competing forces within the thoracic cavity. The first is the elasticity of the lungs themselves and the surface tension of the alveolar fluid. Both pull the lungs inward, away from the chest wall. The lungs are under minimum strain at 1 L of volume. To oppose these forces, the surface tension within the pleural cavity and the chest wall’s elasticity pull the thoracic cage outward, directly opposing the inward pull of the lungs. The minimum strain of the chest wall is at 6 L. At functional residual capacity, the elastic recoils of the lungs and the chest wall are in balance. At this point, there is about 3 L of gas left in the lungs in an average-sized adult.
Therefore, air enters the lungs due to a greater atmospheric pressure and a lower thoracic cavity pressure. We can assume that the atmospheric pressure remains constant, so for air to get in and out of the lungs, we need to adjust the pressures in the lungs and the pleural cavity. For inspiration, thoracic cavity pressure must be less than atmospheric pressure. Whereas, for expiration, the thoracic cavity pressure must be greater than atmospheric pressure.
To help you better understand pressure changes within the lungs, the following video will explain atmospheric pressure, alveolar pressure, and pleural pressure in greater detail. The first four minutes of this video review the two different types of pleura within the pleural cavity. The information presented from the time stamp 4:00-16:40 reviews the various pressures contributing to respiration with a strong focus on pleural pressure in the latter half. Some critical information that you should take away from this learning resource relates to intrapulmonary pressure within the alveoli, plural and atmospheric pressures, and the contributing factors that create negative pleural pressure.
Inspiration
As mentioned in the previous section, for inspiration to occur, thoracic cavity pressure must be less than atmospheric pressure. We can alter alveolar pressure and pleural pressure by changing the volume of the thoracic cavity. This is done by depressing the diaphragm and contracting the external intercostal muscles, as shown in figure 4. This increases the volume in the thoracic cavity, decreasing the pressure according to Boyle’s Law.
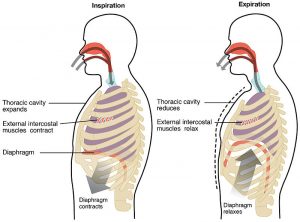
During inspiration, the external intercostals and the diaphragm contract, expanding the chest wall. As a result of this, the lung is further away from its desired position or minimum strain of 1 L, and the chest wall moves closer towards its minimum strain of 6 L. The lung pulls back with more force, which increases the volume in the pleural cavity. Since the volume in the pleural cavity is increased, the pleural decreases and becomes more negative.
At maximal inspiration, the pleural pressure drops to about −12 mmHg. The lung is even further away from its minimum strain of 1 L, so it continues to pull inwards. The chest wall is pulled beyond its minimum strain of 6 L and starts to push inwards as well.
Test Your Knowledge
Real-Life Scenario:
It’s a warm summer evening, and you and your friends decide to head down to the beach to partake in some stargazing. After hours of this relaxing and entertaining activity, you decide to head home. When you sit up, you’re about to inspire, your pleural pressures across your lungs change. What do they change to? Explain why the pleural pressures changed to these new pressures. Hint: How do your lungs move within your thoracic cavity?
The following video is included to help students visualize the pressure changes in the lungs during inspiration. A description of the muscles and their function during inspiration as well as how pressures change to allow for inspiration is the video’s primary focus. The important parts to watch are 9:27 – 16:15, however, the whole video is beneficial. The key takeaways from this video are the discussion of the muscles utilized during respiration, the pressure changes within the thoracic cavity, and how this pressure gradient encourages airflow.
Expiration
Recall that for expiration, thoracic cavity pressure must be greater than atmospheric pressure. In contrast to inspiration, expiration is a passive process that depends entirely upon the lungs’ elasticity, as the lungs desire to resist stretch. During expiration, the intercostal muscles stop contracting, and the diaphragm moves back up to its original place, as shown in figure 4. This decreases the volume in the thoracic cavity, which increases the pressure according to Boyle’s Law. Also, the lungs’ elastic recoil and the chest wall are brought back to balance each other out.
Test Your Knowledge
Real-Life Scenario:
During normal expiration, there is little to no muscle contraction involved. How about forced expiration, when we want to blow more air out than normal? How are we able to force out more air when we breathe? Hint: Think about the process of forced expiration and how it occurs.
The following video is included because it helps students visualize the pressure changes in the lungs during expiration. It does an excellent job of explaining the mechanisms of expiration in greater detail. This video is pretty lengthy, so here are some essential time stamps to look for, the tools that mediate expiration 9:36 – 11:04 and pressure changes during expiration 16:55 – 22:00. Overall, the key takeaways from this video are in relation to the muscles utilized during respiration, the pressure changes within the thoracic cavity, and how airflows down its pressure gradient during expiration.
These have been some big, complex and extremely important concepts covered. Here is a simple activity to verify your understanding of how pleural pressure changes during ventilation. If you find any tough spots, stop here, go back and review the material before moving forward as these are critical concepts.
Lung Volumes
Air in the lungs at different phases of the respiratory cycle is measured in relation to lung volumes and lung capacities. There are five lung volumes, which can be summed up in various combinations to comprise the four lung capacities, all shown in figure 5. Tidal volume (TV) is the amount of air inspired in each breath during quiet normal breathing and is equivalent to approximately 500mL in an average adult. In addition to tidal volume, the inspiratory reserve volume (IRV) is the maximum amount of air that can be inspired that equals about 2000mL in an average adult. Next, expiratory reserve volume (ERV), in addition to tidal volume, is the maximum amount of air that can be expired, which is about 1200 mL in an average adult. Lastly, residual volume (RV) is the amount of air remaining in the lungs after normal expiration equalling about 1200 mL in an average adult. This is the volume of air that cannot be exhaled. Without the residual volume, our alveolar sack would get compressed. This volume cannot be measured directly because it is impossible to empty the lungs fully.
There are four lung capacities, and they are produced by summing up specific lung volumes, all shown in figure 5. First, total lung capacity (TLC) is the total air in the lungs after maximal inspiration. This includes all volumes of the lung: tidal volume, inspiratory and expiratory reserve volume, and residual volume. Next, vital capacity (VC) is the maximum amount of air exhaled after maximal inspiration. It is comprised of tidal volume, inspiratory, and expiratory reserve volumes. Inspiratory capacity (IC) is equivalent to the maximum amount of air inspired after normal expiration. It includes tidal volume and inspiratory reserve volume. Lastly, functional residual capacity (FRC) is the amount of air remaining in the lungs after normal expiration. This capacity is comprised of residual volume and expiratory reserve volume. Test your knowledge on lung volumes and capacities with this fun and interactive quiz!
Did you know that different animals have different lung capacities based on their body sizes and activities? For example, cheetahs have a much higher lung capacity than humans. An increased lung capacity helps provide an adequate amount of oxygen to all of their fast-running muscles. Due to their large size, elephants also have high lung capacities because they must be able to take up oxygen in accordance with their body size.
Test Your Knowledge
Real-Life Scenario:
You went for a run this morning and when you come back to your house you take a seat to catch your breath. You take as big a breath in as you can and push as much air out as you can. What are all of the volumes and capacities associated with this breathing? Hint: Refer to the graph above to help you.
Minute ventilation is the amount of air inspired or expired from a person’s lungs per minute. It can be represented and calculated using the following equation where V̇E is the minute ventilation, VT is the volume per breath and the frequency of breathing is the breath per minute.
[latex]\dot{V}_E=V_T\times(frequency\ of\ breathing)[/latex]
Some of the air within the lungs do not participate in gas exchange because it remains in conducting areas like the trachea, bronchi, and bronchioles. We call this volume the dead space. Therefore, to calculate alveolar ventilation, we can use the following equation where VA equals the alveolar ventilation, VT is the tidal volume, and VDS is the dead space.
[latex]V_A=V_T−V_{DS}[/latex]
On average, dead space is about 150 mL in humans, and the tidal volume of an adult male is about 500 mL. Using the equation above, we can calculate the alveolar ventilation for an average male.
[latex]V_A=V_T−V_{DS}[/latex]
[latex]V_A=500 mL−150 mL[/latex]
[latex]V_A=350 mL[/latex]
Alveolar Pressure, Surface Tension, and Surfactant
Surface tension is a common force that can oppose lung inflation during breathing. The alveoli in our lungs are not all the same size as we have some that are larger and some that are smaller. When we inspire, we essentially inflate our lungs and stretch out our alveoli. The pressure to hold alveoli open is the distending pressure, shown in figure 6. Smaller alveoli have a smaller radius. This means they require a more considerable distending pressure in accordance with the Law of LaPlace, where P is distending pressure, T is surface tension, and r is the radius of the alveoli.
[latex]P=\frac{2T}{r}[/latex]
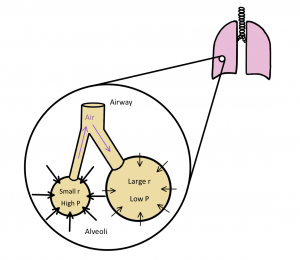
To decrease the surface tension in smaller alveoli and stop a pressure gradient from forming, our epithelial cells in the lungs produce a non-polar phospholipid called surfactant. This creates an air-surfactant interface, shown in figure 7. The air-surfactant interface has two main functions that are critical for breathing. Firstly, surfactant minimizes the work of breathing. This is because there are fewer bonds to break when we want to extend the alveoli. Surfactant is non-polar, meaning it has a fragile attraction for itself and can easily be extended. In addition, surfactant minimizes the pressure differences between alveoli with different radii. Overall, decreasing the surface tension in smaller alveoli.
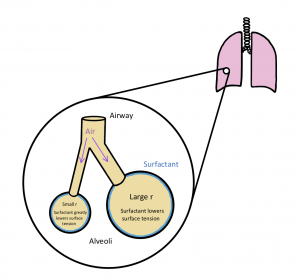
Test Your Knowledge
Clinical Application:
Respiratory Distress Syndrome sometimes occurs in infants. It is caused by decreased production of surfactant. How would this affect their breathing and why? Hint: Recall the two important roles of surfactant in breathing.
The following video is included to help students review surface tension and surfactant fluid. It explains the physics of surfactant fluid and how it allows us to breathe into alveoli of different sizes equally. The Law of LaPlace is highlighted from 3:36-6:16. This law describes the importance of water coating the alveoli in the lungs and how the surface tension created by this water keeps the alveoli from distention and collapse. In addition, the physics of surfactant and its effect within the lungs is showcased from 6:16-11:30. Having a solid understanding of the lungs’ surfactant function will help make future physiology content easier to understand.
Key Takeaways
Consider the following concepts to help guide your studies:
- Respiratory flow is determined by alveolar pressure and airway resistance
- Inspiration and expiration are mediated in part by pleural pressure, which is the pleural cavity pressure, and by atmospheric pressure.
- Alveolar pressure must be negative during inspiration to cause the atmospheric air to be pulled into the smaller radius, as explained by the airflow equation.
- Boyles law dives into the pressure differences between pressure and volume. This inverse relationship can be applied within any breathing scenario.
- Lung capacities are comprised of numerous lung volumes.
- The Law of LaPlace explains the relationship between surface tension and surfactant within the alveolar space.
- Surfactant, a compound within alveoli, has two functions to aid in breathing which are critical.
Subchapter Quiz
The questions below have been created to assess your knowledge within this chapter. You will find five multiple-choice questions which you should try to complete without referring to your notes. Anything you find challenging to complete is a topic you should revisit to help solidify your grasp on the material before moving on. The questions will provide you with responses to your answers to guide your studying.
Media Attributions
- Boyle’s Law © Let's Talk Science is licensed under a All Rights Reserved license
- Pleura and Pressures is licensed under a CC BY (Attribution) license
- Inspiration and Expiration is licensed under a CC BY (Attribution) license
- Lung Volumes and Capacities © Biology LibreTexts is licensed under a CC BY-NC-SA (Attribution NonCommercial ShareAlike) license
- Alveoli Distending Pressure Diagram © Santana Ferreira is licensed under a CC BY (Attribution) license
- Air-Surfactant Interface Diagram © Santana Ferreira is licensed under a CC BY (Attribution) license
The relationship between pressure and volume. At a constant temperature, the volume of a fixed amount of gas is inversely proportional to its pressure.
The difference between the pleural pressure and the alveolar pressure.
The deformation or change in shape and size of material.
The exchange of gas between the alveoli and the atmosphere.