Neuromuscular Communication
The nervous system is the main coordinating center of the human body. It is responsible for appropriately responding to sensory inputs and controlling our internal environment. It also allows for the programming of reflexes and has memory and learning functions. So far, we have talked about the core components of the nervous system and how they communicate with each other. In this subchapter, we will discuss how the nervous system communicates with the rest of the body, specifically the striated muscles of our musculoskeletal system.
Learning Outcomes
In this section you will learn..
- Motor control.
- Definition of a motor unit.
- Understand the neuromuscular junction.
- Distinguish between muscle spindles and Golgi tendon organs (GTOs).
Muscle Spindle – Muscle Stretch Reflex
Tips From Past Students
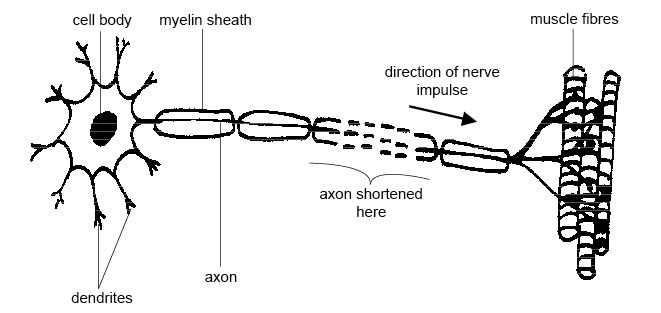
The Stretch Reflex
Another type of reflex is a stretch reflex. In this reflex, when a skeletal muscle is stretched, a muscle spindle receptor is activated. The axon from this receptor structure will cause direct contraction of the muscle. A collateral of the muscle spindle fiber will also inhibit the motor neuron of the antagonist muscles. The reflex helps to maintain muscles at a constant length. A common example of this reflex is the knee jerk that is elicited by a rubber hammer struck against the patellar ligament in a physical exam. This video below explains how the muscle stretch reflex occurs.
The video below will describe to you the receptor properties of muscle spindles. To begin, you’ll get an idea of the location of these muscle spindles, as the creator draws a diagram 0:10-1:50. Important points to note include 2:33- 3:10 when the afferent neurons within the muscles are being described. Also note at 4:30-5:03 the differences between the nuclear bag fibres and the nuclear chain fibres. The video will also describe the important concept of rapid scratch 4:50-6:50.
“Muscle Spindle – Muscle Stretch Reflex” by Animated Anatomy is All Rights Reserved.
Nervous System Control of Muscle Tension[1]
To move an object, referred to as load, the sarcomeres in the muscle fibers of the skeletal muscle must shorten. The force generated by the contraction of the muscle (or shortening of the sarcomeres) is called muscle tension. All of these muscle activities are under the exquisite control of the nervous system. Neural control regulates concentric, eccentric and isometric contractions, muscle fiber recruitment, and muscle tone. A crucial aspect of nervous system control of skeletal muscles is the role of motor units.
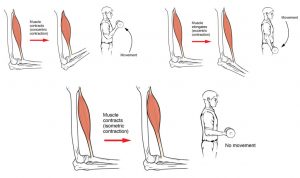
Motor Control[2]
Motor control involves processing sensory input, which is analyzed by the brain to allow for a detailed perception of the external environment. Senses such as vision, somatosensation and audition enable humans to perceive simple stimuli through complex processing. This can all be boiled down to sensory input and motor output. These motor outputs can be as simple as touching a hot pot of boiling water and quickly lifting the hand away in response. Conscious acts require sensory inputs along with many other cognitive processes, which enable us to select the best and appropriate motor response.
There are some terms and components that should be understood are as follows.
The simple definition for volition of this is the power of using one’s will. The motor system needs to coordinate movements to fulfil goals of an organism. These goals are made by the brain and the motor system transforms these goals into real action of the muscle groups involved.
Coordination: Most movements involve the coordination of multiple muscle groups. Different movements require the use of different muscle groups. The motor system must determine the forces and coordination at each individual joint in order to generate a smooth motion.
Proprioception: Before an action is made, the brain must understand the starting point of the body part at hand. For example, raising your hand to ask a question. The motor system needs to know if the hand is starting from rest or sitting on the persons head. The hand will ultimately end up in the same place, however the series of movements to get there varies. Sensory inputs known as proprioceptors tell the motor system the forces applied to the muscle and the length of them.
Sensory Feedback: Sensory information allows for the perform a movement in the desired way. This works along with proprioception. This feedback constantly allows for the correction of movements while they are happening.
Unconscious Processing: All of this is done almost completely autonomically, with the absence of high-order control.
Tips From Past Students
It is important that these terms are understood. Especially sensory feedback. Feedback loops are a major component of the central nervous system and play a large part in many different actions.
Motor Unit
As you have learned, every skeletal muscle fiber must be innervated by the axon terminal of a motor neuron in order to contract. Each muscle fiber is innervated by only one motor neuron. The actual group of muscle fibers in a muscle innervated by a single motor neuron is called a motor unit. The size of a motor unit is variable depending on the nature of the muscle.
A small motor unit is an arrangement where a single motor neuron supplies a small number of muscle fibers in a muscle. Small motor units permit very fine motor control of the muscle. The best example in humans is the small motor units of the extraocular eye muscles that move the eyeballs. There are thousands of muscle fibers in each muscle, but every six or so fibers are supplied by a single motor neuron, as the axons branch to form synaptic connections at their individual NMJs. This allows for exquisite control of eye movements so that both eyes can quickly focus on the same object. Small motor units are also involved in the many fine movements of the fingers and thumb of the hand for grasping, texting, etc.
A large motor unit is an arrangement where a single motor neuron supplies a large number of muscle fibers in a muscle. Large motor units are concerned with simple, or “gross,” movements, such as powerfully extending the knee joint. The best example is the large motor units of the thigh muscles or back muscles, where a single motor neuron will supply thousands of muscle fibers in a muscle, as its axon splits into thousands of branches.
There is a wide range of motor units within many skeletal muscles, which gives the nervous system a wide range of control over the muscle. The small motor units in the muscle will have smaller, lower-threshold motor neurons that are more excitable, firing first to their skeletal muscle fibers, which also tend to be the smallest. Activation of these smaller motor units, results in a relatively small degree of contractile strength (tension) generated in the muscle. As more strength is needed, larger motor units, with bigger, higher-threshold motor neurons are enlisted to activate larger muscle fibers. This increasing activation of motor units produces an increase in muscle contraction known as recruitment. As more motor units are recruited, the muscle contraction grows progressively stronger. In some muscles, the largest motor units may generate a contractile force of 50 times more than the smallest motor units in the muscle. This allows a feather to be picked up using the biceps brachii arm muscle with minimal force, and a heavy weight to be lifted by the same muscle by recruiting the largest motor units.
When necessary, the maximal number of motor units in a muscle can be recruited simultaneously, producing the maximum force of contraction for that muscle, but this cannot last for very long because of the energy requirements to sustain the contraction. To prevent complete muscle fatigue, motor units are generally not all simultaneously active, but instead some motor units rest while others are active, which allows for longer muscle contractions. The nervous system uses recruitment as a mechanism to efficiently utilize a skeletal muscle.
The Length-Tension Range of a Sacromere
When a skeletal muscle fiber contracts, myosin heads attach to actin to form cross-bridges followed by the thin filaments sliding over the thick filaments as the heads pull the actin, and this results in sarcomere shortening, creating the tension of the muscle contraction. The cross-bridges can only form where thin and thick filaments already overlap, so that the length of the sarcomere has a direct influence on the force generated when the sarcomere shortens. This is called the length-tension relationship.
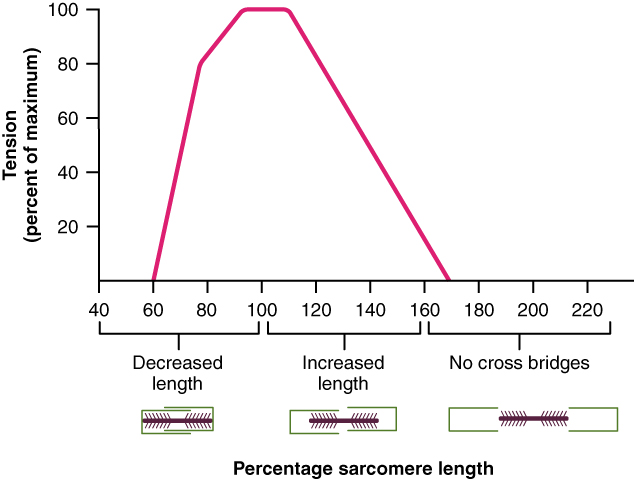
The ideal length of a sarcomere to produce maximal tension occurs at 80 percent to 120 percent of its resting length, with 100 percent being the state where the medial edges of the thin filaments are just at the most-medial myosin heads of the thick filaments, as shown in figure 2.
This length maximizes the overlap of actin-binding sites and myosin heads. If a sarcomere is stretched past this ideal length (beyond 120 percent), thick and thin filaments do not overlap sufficiently, which results in less tension produced. If a sarcomere is shortened beyond 80 percent, the zone of overlap is reduced with the thin filaments jutting beyond the last of the myosin heads and shrinks the H zone, which is normally composed of myosin tails.
Eventually, there is nowhere else for the thin filaments to go and the amount of tension is diminished. If the muscle is stretched to the point where thick and thin filaments do not overlap at all, no cross-bridges can be formed, and no tension is produced in that sarcomere. This amount of stretching does not usually occur, as accessory proteins and connective tissue oppose extreme stretching.
The Frequency of Motor Neuron Stimulation
A single action potential from a motor neuron will produce a single contraction in the muscle fibers of its motor unit. This isolated contraction is called a twitch. A twitch can last for a few milliseconds or 100 milliseconds, depending on the muscle type. The tension produced by a single twitch can be measured by a myogram, an instrument that measures the amount of tension produced over time, as seen in figure 3. Each twitch undergoes three phases.
The first phase is the latent period, during which the action potential is being propagated along the sarcolemma and Ca++ ions are released from the SR. This is the phase during which excitation and contraction are being coupled but contraction has yet to occur. Thecontraction phase occurs next. The Ca++ ions in the sarcoplasm have bound to troponin, tropomyosin has shifted away from actin-binding sites, cross-bridges formed, and sarcomeres are actively shortening to the point of peak tension. The last phase is the relaxation phase, when tension decreases as contraction stops. Ca++ ions are pumped out of the sarcoplasm into the SR, and cross-bridge cycling stops, returning the muscle fibers to their resting state.
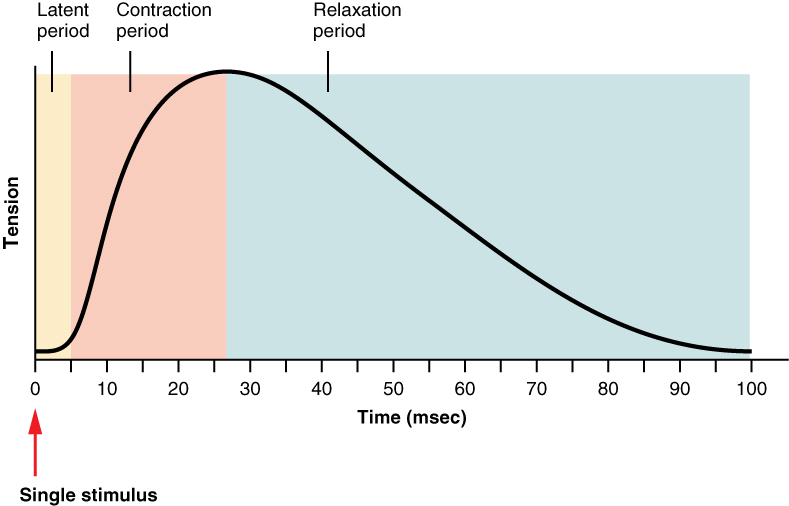
To reiterate, s single muscle twitch has a latent period, a contraction phase when tension increases, and a relaxation phase when tension decreases. During the latent period, the action potential is being propagated along the sarcolemma. During the contraction phase, Ca++ ions in the sarcoplasm bind to troponin, tropomyosin moves from actin-binding sites, cross-bridges form, and sarcomeres shorten. During the relaxation phase, tension decreases as Ca++ ions are pumped out of the sarcoplasm and cross-bridge cycling stops.
Although a person can experience a muscle “twitch,” a single twitch does not produce any significant muscle activity in a living body. A series of action potentials to the muscle fibers is necessary to produce a muscle contraction that can produce work. Normal muscle contraction is more sustained, and it can be modified by input from the nervous system to produce varying amounts of force; this is called a graded muscle response. The frequency of action potentials (nerve impulses) from a motor neuron and the number of motor neurons transmitting action potentials both affect the tension produced in skeletal muscle.
The rate at which a motor neuron fires action potentials affects the tension produced in the skeletal muscle. If the fibers are stimulated while a previous twitch is still occurring, the second twitch will be stronger. This response is called wave summation, because the excitation-contraction coupling effects of successive motor neuron signaling is summed, or added together, shown in figure 4a. At the molecular level, summation occurs because the second stimulus triggers the release of more Ca++ ions, which become available to activate additional sarcomeres while the muscle is still contracting from the first stimulus. Summation results in greater contraction of the motor unit.
If the frequency of motor neuron signaling increases, summation and subsequent muscle tension in the motor unit continues to rise until it reaches a peak point. The tension at this point is about three to four times greater than the tension of a single twitch, a state referred to as incomplete tetanus. During incomplete tetanus, the muscle goes through quick cycles of contraction with a short relaxation phase for each. If the stimulus frequency is so high that the relaxation phase disappears completely, contractions become continuous in a process called complete tetanus, seen in figure 4b.
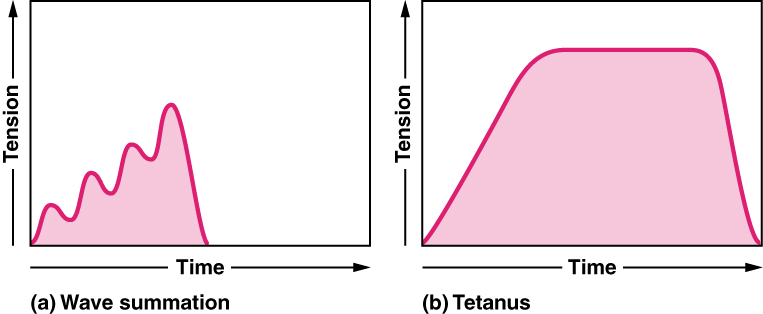
During tetanus, the concentration of Ca++ ions in the sarcoplasm allows virtually all of the sarcomeres to form cross-bridges and shorten, so that a contraction can continue uninterrupted (until the muscle fatigues and can no longer produce tension).
Treppe Phenonemon
When a skeletal muscle has been dormant for an extended period and then activated to contract, with all other things being equal, the initial contractions generate about one-half the force of later contractions. The muscle tension increases in a graded manner that to some looks like a set of stairs. This tension increase is called treppe, a condition where muscle contractions become more efficient. It’s also known as the “staircase effect”, shown in figure 5.
It is believed that treppe results from a higher concentration of Ca++ in the sarcoplasm resulting from the steady stream of signals from the motor neuron. It can only be maintained with adequate ATP.
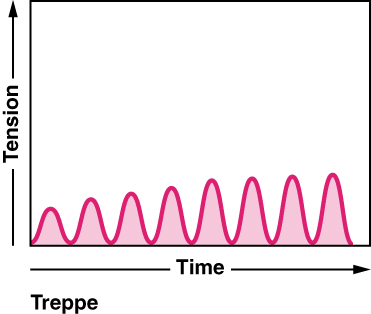
Muscle Tone
Skeletal muscles are rarely completely relaxed, or flaccid. Even if a muscle is not producing movement, it is contracted a small amount to maintain its contractile proteins and produce muscle tone. The tension produced by muscle tone allows muscles to continually stabilize joints and maintain posture.
Muscle tone is accomplished by a complex interaction between the nervous system and skeletal muscles that results in the activation of a few motor units at a time, most likely in a cyclical manner. In this manner, muscles never fatigue completely, as some motor units can recover while others are active.
The absence of the low-level contractions that lead to muscle tone is referred to as hypotonia or atrophy, and can result from damage to parts of the central nervous system (CNS), such as the cerebellum, or from loss of innervations to a skeletal muscle, as in poliomyelitis. Hypotonic muscles have a flaccid appearance and display functional impairments, such as weak reflexes. Conversely, excessive muscle tone is referred to as hypertonia, accompanied by hyperreflexia (excessive reflex responses), often the result of damage to upper motor neurons in the CNS. Hypertonia can present with muscle rigidity (as seen in Parkinson’s disease) or spasticity, a phasic change in muscle tone, where a limb will “snap” back from passive stretching (as seen in some strokes).
The Neuromuscular Junction AL[3]
Skeletal muscle contraction occurs when calcium ions are released from internal stores in response to a neural signal. Skeletal muscle fibres are controlled by motor neurons, which allow for the conduction of a signal from the brain or spinal cord to the muscle.
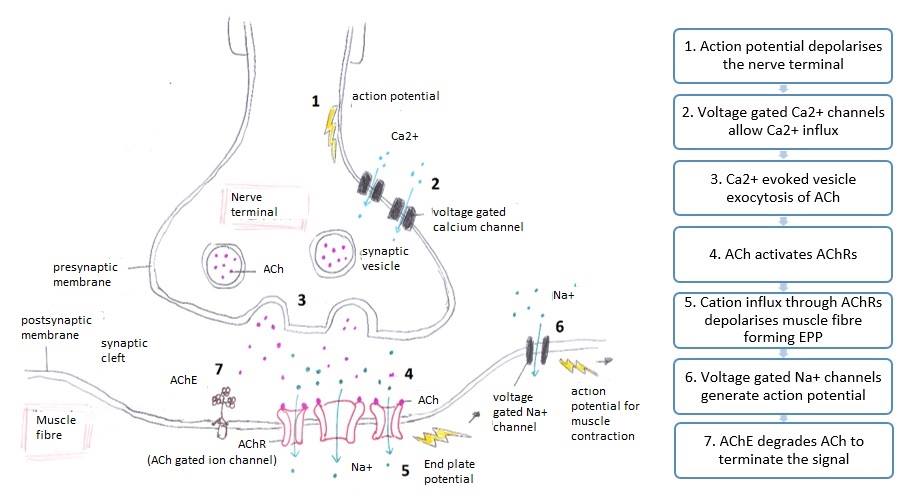
The sequence involved in contraction of skeletal muscle:
- The action potential travels down the neuron to the presynaptic terminal.
- Voltage-gated calcium channels open and Ca2+ ions travel from the extracellular fluid into the presynaptic neuron.
- This influx of Ca2+ causes neurotransmitter vesicles (often acetylcholine) to travel towards the axon terminal. The neurotransmitter vesicles fuse with the cell membrane and the neurotransmitter is released into the synaptic cleft.
- Acetylcholine will bind to nicotinic acetylcholine receptors which are ligand-gated. The binding of neurotransmitters causes the ligand-gated channels to open.
- Sodium ions flow through the open channels into the muscle, which leads to a large electrochemical gradient across the membrane and there is a local depolarization of the motor end plate.
- This transduction opens voltage-gated sodium channels, in which sodium flows in and potassium flows out of the muscle. This electrochemical gradient leads to a local depolarization of the motor end plate, causing an action potential, leading to contraction.
- Acetylcholine is displaced from its receptor and is degraded by acetylcholinesterase. The breakdown products are recycled to the presynaptic terminal and can be used to synthesize new acetylcholine.
Tips From Past Students
It may seem daunting to memorize the steps of the neuromuscular junction, however, it will be much easier to visualize the steps in order to truly understand what is happening. This is a concept that will likely appear on examinations, so it is crucial that it is properly understood.
Something that students may forget to study, is when channels are voltage-gated or ligand-gated. In this case, the calcium channels are voltage-gated and the sodium channels are ligand-gated.
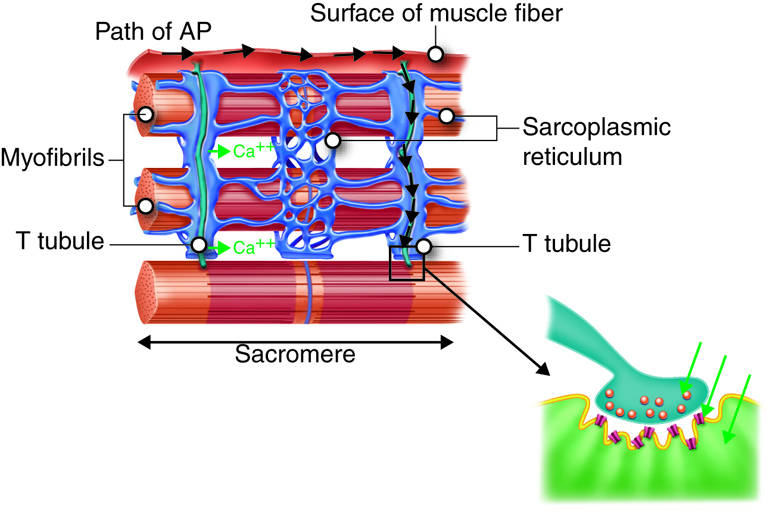
Ever wonder how select autoimmune diseases can affect motor control? Lambert-Eaton Myasthenic Syndrome (LEMS), is an autoimmune disorder that causes muscle weakness in the limbs. It is caused by antibodies attacking voltage-gated calcium channels in the neuromuscular junction. Often this disease is referred to as paraneoplastic syndrome, as it is a consequence of cancer. Most patients are more affected in the lower limbs, making novel tasks extremely challenging.
Antibodies against the voltage-gated calcium channels prevent a sufficient amount of calcium from being released into the nerve. This consequently leads to less acetylcholine being released. This decreases the effectiveness of the neuromuscular junction and makes it very difficult to contract muscle.
This disease is diagnosed with nerve conduction studies and electromyography. Nerve conduction studies analyzes compound motor action potentials (CMAPs) of the muscles that are affected. As impulses are introduced to the nerve, CMAP amplitudes will decrease as acetylcholine levels drop. If a patient has LEMS, the shock will unlikely be able to transmit an impulse from the nerve to the muscle.
Somatosensation, Muscle Spindles, Golgi Tendon Organs[4]
In proprioception, proprioceptive and kinesthetic signals travel through myelinated afferent neurons running from the spinal cord to the medulla. Neurons are not physically connected, but communicate via neurotransmitters secreted into synapses or “gaps” between communicating neurons. Once in the medulla, the neurons continue carrying the signals to the thalamus.
Muscle spindles are stretch receptors that detect the amount of stretch, or lengthening of muscles. Related to these are Golgi tendon organs, which are tension receptors that detect the force of muscle contraction. Proprioceptive and kinesthetic signals come from limbs. Unconscious proprioceptive signals run from the spinal cord to the cerebellum, the brain region that coordinates muscle contraction, rather than to the thalamus, like most other sensory information.
Baroreceptors detect pressure changes in an organ. They are found in the walls of the carotid artery and the aorta where they monitor blood pressure, and in the lungs where they detect the degree of lung expansion. Stretch receptors are found at various sites in the digestive and urinary systems.
Tips From Past Students
Muscle spindles and Golgi tendon organs are both receptors that are very important, however they are not the same, meaning that they are responsible for different tasks. It is crucial to be able to distinguish between them. Muscle spindles are responsible for detecting the amount of stretch, whereas GTOs are tension receptors.
Motor Responses[5]
The general function of the somatic nervous system is to control skeletal muscle. It is important to distinguish the difference between voluntary and involuntary muscle movement. When the nervous system receives input from somatic senses about the external environment, there is a voluntary muscle response. This notion of voluntary movement can be explained by the fact that it is performed under conscious control. There are parts of the somatic nervous system that involve muscle movement without conscious control. Breathing is a prime example of this.
Cortical Responses
Let’s start with sensory stimuli that have been registered through receptor cells and the information relayed to the CNS along ascending pathways. In the cerebral cortex, the initial processing of sensory perception progresses to associative processing and then integration in multimodal areas of cortex. These levels of processing can lead to the incorporation of sensory perceptions into memory, but more importantly, they lead to a response. The completion of cortical processing through the primary, associative, and integrative sensory areas initiates a similar progression of motor processing, usually in different cortical areas. While the sensory cortical areas are located in the occipital, temporal, and parietal lobes, motor functions are largely controlled by the frontal lobe.
The Function of Nervous Tissue [6]
Tips From Past Students
This diagram is a great summary of what we’ve learned so far. This pathway is important to understand not just as a concept, but as a whole.
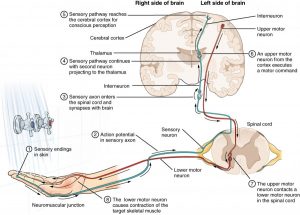
Key Terms Exercise
Key Takeaways
Consider the following concepts to help guide your studies:
- Stretch reflexes, including the mechanism of action of muscle spindles, nuclear chain fibres and nuclear bag fibres.
- The different types of movement caused by the relaxation or contraction of motor units.
- The components of a motor unit, and how an increased stretch of contraction is the result of more motor unit recruitment.
- The importance of wave summation in the production of a stronger muscle contraction.
- The difference in the roles of somatosensation, muscle spindles and the Golgi tendon organs.
Subchapter Quiz
The questions below can be used to assess your knowledge within this chapter. There are five multiple-choice questions that you should attempt without referring to your notes. The questions will provide you with responses to your answers to guide your studying but should not be used as your only resource.
Media Attributions
- Neuron Diagram © Ruth Lawson is licensed under a CC0 (Creative Commons Zero) license
- Muscle Contractions © OpenStax College is licensed under a CC BY (Attribution) license
- Line Graph © Boundless is licensed under a CC BY-SA (Attribution ShareAlike) license
- Myograph © OpenStax College is licensed under a CC BY (Attribution) license
- Two Graphs © OpenStax College is licensed under a CC BY (Attribution) license
- Treppe © OpenStax College is licensed under a CC BY (Attribution) license
- NMJ Signalling © Wikipedia is licensed under a Public Domain license
- Muscle Fibers and NM Junction © Open Learning Initiative is licensed under a CC BY-NC-SA (Attribution NonCommercial ShareAlike) license
- Testing the Water © OpenStax College is licensed under a CC BY (Attribution) license
- OpenStax College (n.d.). Nervous System Control of Muscle Tension. Retrieved April 10, 2021 from https://courses.lumenlearning.com/ap1/chapter/nervous-system-control-of-muscle-tension/ ↵
- Knierim, J. (20 October 2020). Chapter 1: Motor Units and Muscle Receptors. Retrieved April 10, 2021 from https://nba.uth.tmc.edu/neuroscience/s3/chapter01.html ↵
- Open Learning Initiative (n.d.). Neuromuscular Junctions and Muscle Contractions. Retrieved April 10, 2021 from https://courses.lumenlearning.com/cuny-csi-ap-1/chapter/neuromuscular-junctions-and-muscle-contractions/ ↵
- OER Commons (n.d.). Somatosensation. Retrieved April 10, 2021 from https://www.oercommons.org/courseware/module/15122/overview ↵
- OpenStax College (n.d.). Motor Responses. Retrieved April 10, 2021 from https://courses.lumenlearning.com/ap1/chapter/motor-responses/ ↵
- OpenStax College (n.d.). The Function of Nervous Tissue. Retrieved April 10, 2021 from https://courses.lumenlearning.com/ap1/chapter/the-function-of-nervous-tissue/ ↵
Stretch receptors in the muscle that detect changes in its length.
[of a neuron] A sensory neuron that carries information from sensory stimuli to the brain.
A type of intrafusal muscle fibre that make up the muscle spindle; are dynamic in nature.
A type of intrafusal muscle fibre that makes up the muscle spindle; are static in nature.
The motor neuron and its associated skeletal muscle fibres, which work together to coordinate movement of skeletal muscles.
The ability of the body to perceive its position in space and the position of each body part relative to others.
A graphical representation of muscle activity.
A sensory organ that receives information from the tendons; senses changes in tension exerted by muscles.